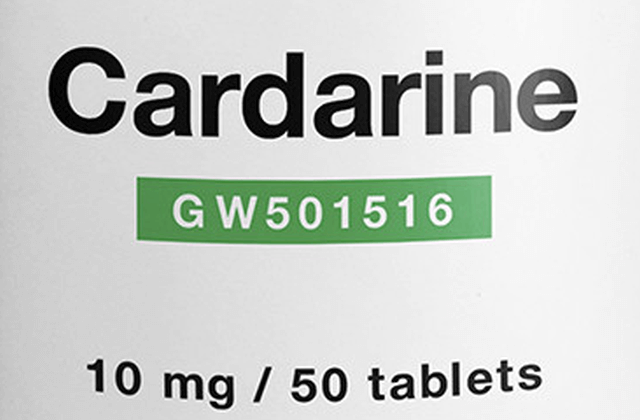
Estimated reading time: 12 minutes
Table of contents
Introduction
In 1990, researchers published the discovery of a new nuclear receptor—named the peroxisome proliferator-activated receptor ((PPAR)—in the journal Nature [1]. The name refers to the proliferation of peroxisomes, organelles of cells with a prominent metabolic function, upon activation of these receptors. This discovery would finally help solve the mystery of how a class of drugs named fibrates worked: they acted on this receptor. Fibrates had been synthesized since the 1950s and are still prescribed today for the treatment of hypertriglyceridemia. Hypertriglyceridemia is a condition in which triglycerides in the blood are elevated. The treatment of this condition is aimed at reducing the risk of pancreatitis and atherosclerotic cardiovascular disease [2].
The receptor reported in Nature later turned out to be one of three receptors belonging to the same group: PPARα, PPARδ (also known as PPARβ) and PPARγ. These are pronounced as PPAR alpha, delta and gamma, respectively. The endogenous ligands (molecules that bind to a receptor) for the PPARs are a variety of (polyunsaturated) fatty acids and their derivatives [3]. As such, they can be viewed as lipid sensors. Given that PPARs are nuclear receptors, and thus regulate gene transcription, the availability of fatty acids is able to regulate gene transcription through activation of these receptors. Briefly, PPARα mainly plays a role in regulation of lipid metabolism, PPARδ in glucose metabolism and muscle’s oxidative capacity, and PPARγ in the formation of new fat cells, fatty acid uptake and storage, and consequently insulin sensitivity [4]. Overall, activation of these receptors also shows antiatherogenic properties and affects inflammatory processes.
In addition to the widespread and long-standing use of fibrates, glitazones (also known as thiazolidinediones) are another class of widely prescribed pharmaceuticals that act on PPARs. More specifically, they act on PPARγ. Their hallmark is the positive effect on insulin sensitivity. As a result of this, they’re prescribed in the treatment of type 2 diabetes.
To date, however, there’s not a single approved drug that selectively acts on PPARδ. That’s not to say that the pharmaceutical industry hasn’t been interested in this receptor subtype: a tremendous amount of research has been done in this area. In this article I will focus on a candidate drug that acts on this receptor and made it into clinical trials: cardarine (GW501516). While the clinical trials showed promising results, two rodent studies that screened for carcinogenicity of the compound casted a dark shadow on it.
Cardarine (GW501516) is a PPARδ agonist developed by GlaxoSmithKline (GSK)
Cardarine was developed by Glaxo Wellcome in the 1990s, nowadays known as GlaxoSmithKline (GSK) after they merged with SmithKline Beecham. Hence the GW prefix of their compound names such as GW501516.
GSK found cardarine to be a selective agonist of PPARδ and their animal experiments showed some interesting results: a partial correction of hyperinsulinemia, an increase in HDL cholesterol, and a decrease in VLDL and LDL cholesterol, and fasting triglycerides [5]. Moreover, their research suggested that it increased reverse cholesterol transport, i.e. cholesterol efflux. Reverse cholesterol transport is the process through which HDL particles are thought to protect arteries from deteriorating. Importantly, while GW501516 demonstrated an increase in cholesterol efflux, the PPARα and PPARγ agonists they tested did not. Suggesting a PPARδ-specific effect.
Obviously, GSK had enough reason to take the drug into clinical trials.
Cardarine (GW501516) made it into clinical trials
The first clinical trial was published in 2006 [6]. A total of 24 healthy male subjects were randomized to a placebo (n=6), cardarine 2.5 mg daily (n=9) or cardarine 10 mg daily (n=9) for a total of 2 weeks. The participants were housed in their research facility under hospitalized care and were sedentary and prohibited from performing rigorous physical activity. Additionally, they received a standardized diet (about 2000 kcal daily, 26% of which was fat). Keep this mind, as the research setting is important for interpretation of the results.
They measured a lipid panel including total, LDL, and HDL cholesterol, triglycerides and ApoA1. While most of you are most likely familiar with the first few values, I suspect some of you reading this aren’t familiar with ApoA1. ApoA1 is a so-called apolipoprotein. An apolipoprotein is a protein that’s associated with lipoprotein such as LDL and HDL cholesterol. ApoA1 is associated with HDL cholesterol, although it’s also associated with Chylomicrons. Chylomicrons are responsible for the transport of lipids absorbed by the gastrointestinal tract to the rest of the body.
No statistically significant differences were found for total cholesterol, and LDL cholesterol. HDL cholesterol showed an increase in both cardarine groups compared to placebo. However, the difference was mainly due to a decrease in HDL cholesterol that was observed in the placebo group. This is possibly the result of the 2-week sedentary lifestyle, and perhaps the standardized diet, that the subjects were subject to. In other words, one could argue that cardarine counteracts the small decrease in HDL cholesterol that’s observed when switching from an active to a sedentary lifestyle. Triglycerides showed a significant within group difference in the 10 mg cardarine group. However, this decrease was not significant compared to placebo. The decrease was relatively big (from 1.04 to 0.76 mmol/L), so this might have been a type II error. Or in other words: their sample size was too small to detect it. Finally, ApoA1 showed a significant decrease in the 10 mg cardarine group only compared to placebo. However, just as with the HDL cholesterol increase, this was the result of a decrease of this value in the placebo group rather than an increase in the cardarine group. In fact, it remained unchanged in this group (116 mmol/L both at baseline as well as the end of the study).
They also performed a ‘fat feeding study’. Meaning that they gave a fatty meal at the beginning and the end (or actually day 13) of the study while measuring the triglyceride response in the 24 hours after the meal. A higher triglyceride response suggests tissue uptake of fat is diminished, whereas a lower triglyceride response suggests tissue uptake of fat is enhanced. The latter being the desired outcome. Whereas the placebo group saw a 36.1 % increase in the triglyceride response (i.e. the 24-hour area under the curve of the triglyceride concentration), the 2.5 mg group saw a 15.3 % decrease (not statistically significant compared to placebo), and the 10 mg group saw a 25.4 % decrease (statistically significant compared to placebo).
Finally, blood pressure remained unchanged in all groups, and no adverse events (including liver and muscle responses) were reported.
The results of these blood measurements, combined with data obtained from cell culture and animal studies, led the authors to conclude that PPARδ affects enzymes critical to fatty acid oxidation, and they make the connection with what’s observed in response to exercise.
The next clinical trial was set out “To test the hypothesis that lipid lowering can be achieved by an agonist to PPARδ through enhancement of fatty acid oxidation without adverse effects on oxidative stress (…)” [7]. Just as the previous trial, this was a 2-week study at their research facility. Subjects were randomized to a placebo (n=6), cardarine (n=6), or a PPARα agonist (GW590735, n=6). The cardarine group received 10 mg daily.
An important difference between the subjects in this study and the previous one, is that these subjects were apparently healthy but abdominally obese witih moderate dyslipidemia. Keep that in mind when interpreting the results.
In addition to a lipid panel consisting of nonesterified fatty acids (NEFA), triglycerides, total, LDL and HDL cholesterol, and ApoB, the researchers also measured:
- markers of glucose homeostasis (glucose, insulin, and consequently HOMA-IR; a number supposed to represent insulin resistance)
- markers of liver damage (GGT, AST, and ALT)
- β-hydroxybutyric acid (as a marker of hepatic fatty acid oxidation)
- urinary F2-isoprostanes (as a marker of whole-body oxidative stress)
- liver fat
Glucose, insulin, HOMA-IR, NEFA, triglycerides, total cholesterol, LDL cholesterol, ApoB, β-hydroxybutyric acid, urinary F2-isoprostanes, liver fat, and GGT all showed statistically significant decreases in the group receiving cardarine compared to baseline. However, compared to placebo, only the changes in insulin, HOMA-IR, NEFA, and liver fat were statistically significant. Because of the small group sizes, a type II error might’ve occurred for some of the values. (Not finding a statistically significant difference while there’s an actual true difference.) The NEFA and liver fat decreases in particular were quite pronounced, by 40% and 20%, respectively.
A 6-hour meal tolerance test was also conducted. This meal contained a small amount of radiolabeled palmitate (a fatty acid). This means that some of the carbon atoms in the meal were of a specific isotope (carbon-13). When these fatty acids are being oxidized by the body, these isotopes will be exhaled as a part of carbon dioxide. This, in turn, can be measured. The researchers could thus measure if cardarine affected fatty acid oxidation after a meal. Which it certainly seemed to do, since the postprandial area under the curve of labeled NEFA was lower, and the relative abundance of these labeled carbon atoms in expired breath increased in the group receiving cardarine.
With an increase in skeletal muscle fatty acid oxidation speculated as lying at the root of these positive effects on metabolic abnormalities, cardarine appears to be an interesting compound for the treatment of hyperlipidemia, liver fat accumulation, obesity and insulin resistance.
In keeping with these results, a later small-scale trial further explored the effects on (apo)lipoproteins at a dosage of 2.5 mg daily [8]. Interestingly, a later paper, which described two trials, including a large-scale 12-week trial with 268 participants, was published in 2012 [9]. It showed more of the same, but the interesting part is that the paper concludes with: “Long-term preclinical safety studies have precluded further development of GW501516 for dyslipidemia indications.” So what exactly are they talking about here? Obviously GSK was very interested in bringing this drug to market, given that it entered phase II trials with a large number of participants.
The 2-year rodent carcinogenicity bioassays showed worrying results
The pharmaceutical industry is expected, or pretty much mandated, to explore the carcinogenicity of new pharmaceuticals they intend to bring to market. Historically the FDA and it’s European equivalent the European Medicines Agency (EMA) required the 2-year rodent bioassay for this. Which boils down to giving mice and rats the substance for 2 years, and then assessing the cancers that have arised. This has been done for decades and there’s a wealth of data on the most commonly used rat strains for this type of studies. The bioassay has good sensitivity and specificity [10]. Meaning that, if a substance is carcinogenic, there’s a good chance this bioassay will demonstrate this. Similarly, if a substance is not carcinogenic, there’s also a good chance this bioassay will demonstrate that.
The full data of the 2-year rodent bioassays have not been published by GSK, but a brief summary of their result has [11, 12].
In the rat study, male rats were given 0, 5, 20, or 40 mg/kg/day, whereas female rats were given 0, 3, 10 or 20 mg/kg/day. The results showed increased mortality in females at all doses, with neoplasms considered related to cardarine in various tissues at all dosages, namely:
- Hepatocellular adenoma at >10 mg/kg/day
- Urinary bladder transitional cell carcinoma in males at 20 and 40 mg/kg day
- Thyroid follicular cell adenoma at >3 mg/kg/day and carcinoma in males at >20 mg/kg/day
- Tongue squamous cell papilloma in males at 5 and 40 mg/kg/day
- Stomach squamous cell papilloma in males at >5 mg/kg/day and a female at 20 mg/kg/day, and carcinoma in a male at 40 mg/kg/day and a female at 3 mg/kg/day
- Skin inverted squamous cell papilloma in males at >5 mg/kg/day and females at 3 or 20 mg/kg/day
- Harderian glands adenoma in males at >5 mg/kg/day and adenocarcinoma in a male at 40 mg/kg/day
- Testes interstitial cell adenoma at 40 mg/kg/day
- Ovary Sertoli cell adenoma at >10 mg/kg/day
- Uterus polyp and endometrial adenocarcinoma at >3 mg/kg/day
In the mice study, mice were given 0, 10, 30, 60 or 80 mg/kg/day. The results showed increased mortality at doses >30 mg/kg/day and neoplasms considered related to cardarine in various tissues, namely:
- Hepatocellular carcinoma at >30 mg/kg/day and adenoma at >10 mg/kg/day
- Stomach squamous cell carcinoma at all dosages
- Combined squamous cell tumours at all dosages (squamous cell papilloma and carcinoma, and keratoacanthoma)
If you’re a pharmaceutical company, these aren’t results you’re waiting for and I highly doubt the FDA or EMA would approve a drug with such worrying results for an indication such as dyslipidemia. Of course, the details of the study haven’t been published, but if anything, GSK would have favored the results in the opposite direction. They didn’t hijack their own drug by fucking up the 2-year rodent bioassay that I’m sure they’ve done countless times over the past few decades. It’s pretty evident why GSK has discontinued the development of this drug. An important question remains: is this carcinogenicity specific to the compound used, or to PPARδ agonists in general?
As a final note, given these results, it seems very unwise to consume this drug chronically.
References
- Issemann, Isabelle, and Stephen Green. “Activation of a member of the steroid hormone receptor superfamily by peroxisome proliferators.” Nature 347.6294 (1990): 645-650.
- Simha V. Management of hypertriglyceridemia BMJ 2020; 371 :m3109 doi:10.1136/bmj.m3109
- Grimaldi, P. A. “Lipid sensing and lipid sensors.” Cellular and Molecular Life Sciences 64.19 (2007): 2459-2464.
- Montaigne, David, Laura Butruille, and Bart Staels. “PPAR control of metabolism and cardiovascular functions.” Nature Reviews Cardiology (2021): 1-15.
- Oliver, William R., et al. “A selective peroxisome proliferator-activated receptor δ agonist promotes reverse cholesterol transport.” Proceedings of the national academy of sciences 98.9 (2001): 5306-5311.
- Sprecher, Dennis L., et al. “Triglyceride: high-density lipoprotein cholesterol effects in healthy subjects administered a peroxisome proliferator activated receptor δ agonist.” Arteriosclerosis, thrombosis, and vascular biology 27.2 (2007): 359-365.
- Risérus, Ulf, et al. “Activation of peroxisome proliferator–activated receptor (PPAR) δ promotes reversal of multiple metabolic abnormalities, reduces oxidative stress, and increases fatty acid oxidation in moderately obese men.” Diabetes 57.2 (2008): 332-339.
- Ooi, Esther MM, et al. “Mechanism of action of a peroxisome proliferator-activated receptor (PPAR)-δ agonist on lipoprotein metabolism in dyslipidemic subjects with central obesity.” The Journal of Clinical Endocrinology & Metabolism 96.10 (2011): E1568-E1576.
- Olson, Eric J., et al. “Lipid effects of peroxisome proliferator-activated receptor-δ agonist GW501516 in subjects with low high-density lipoprotein cholesterol: characteristics of metabolic syndrome.” Arteriosclerosis, thrombosis, and vascular biology 32.9 (2012): 2289-2294.
- Suarez-Torres, Jose D., Fausto A. Jimenez-Orozco, and Carlos E. Ciangherotti. “The 2-year rodent bioassay in drug and chemical carcinogenesis testing: sensitivity, according to the framework of carcinogenic action.” Toxicology mechanisms and methods 30.6 (2020): 462-475.
- Geiger, L. E., et al. “Rat carcinogenicity study with GW501516, a PPAR delta agonist.” The Toxicologist 108.1 (2009): 895.
- Newsholme, S. J., et al. “Mouse carcinogenicity study with gw501516, a PPAR delta agonist.” The Toxicologist 108.1 (2009): 896.
About the author
Peter Bond is a scientific author with publications on anabolic steroids, the regulation of an important molecular pathway of muscle growth (mTORC1), and the dietary supplement phosphatidic acid. He is the author of several books in Dutch and English, including Book on Steroids and Bond's Dietary Supplements.
37 replies
Loading new replies...
Join the full discussion at the MESO-Rx →