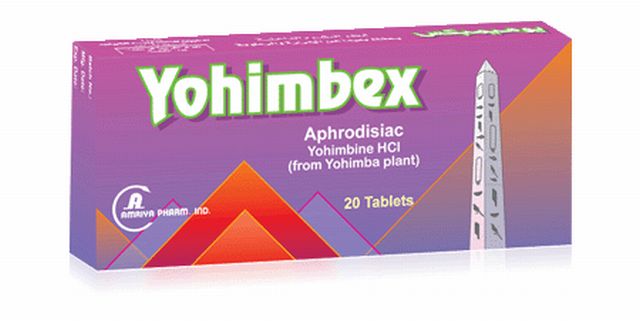
Loss of body fat has become an obsession for Western society. Accordingly, overweight and obesity are associated with high risks for various pathophysiological conditions and a lower mortality rate. Approaches to body fat loss vary according to genetic and environmental factors. Moreover, regulation of both energy intake and energy expenditure can be moderated in several ways. The autonomic nervous system contributes to maintenance of homeostasis in the body by way of the sympathetic nervous system. This system stimulates energy mobilization and utilization in many tissues. Therefore, manipulation of the sympathetic nervous system may change body composition. This article will examine the role of fat tissue metabolism, the sympathetic nervous system, and a pharmaceutical approach to fat loss.
The physiological role of the sympathetic nervous system (SNS) is a major target of study for modulation of body weight and composition. The principal role players in the SNS are hormones called catecholamines and the adrenergic receptors (adrenoceptors) upon which they act. Aside from insulin, the catecholamines are the primary regulators of fat breakdown in cells by way of stimulation of adrenoceptors on the cell membrane. Activation of these adrenoceptors produces differing responses in the cell depending on receptor type, subtype and tissue. Two types of adrenoceptors, alpha- and beta-adrenoceptors, differentially stimulate or inhibit lipolysis and provide for dual control mechanisms within the fat cell.
A host of drugs that manipulate the SNS to reduce body fat have appeared and disappeared in the last few decades. Bryan Haycock published an article on MESO-Rx examining the role of beta-adrenergic receptors in fat loss and diet drugs that increase lipolysis (Pharmacological Approaches to Fat Loss: Targeting Beta-Adrenergic Receptors) at the b -receptor and post-receptor levels. This article will examine the role of alpha-adrenoceptors and an alternative pharmaceutical approach to fat loss.
The first part of this article will discuss adrenoceptors and their function so that readers may comprehend the dual adrenergic regulation of lipolysis. The lipolytic process was discussed in Bryan’s article (see above), but will be summarized here to acquaint those who have not read Bryan’s article. The second installment of the article will examine the role of alpha2-adrenoceptors in adipose and other tissues and the ways they influence regulation of lipolysis. Finally, we will see how yohimbine, an alpha2-adrenoceptor antagonist can be of use in the pharmacological approach to fat loss.
Lipolysis Revisited
Adipose tissue, commonly called body fat, serves several functions in the body such as in storage and provision of energy, insulation, and mechanical support as in the sole of the foot or in the palm of the hand. The majority of human adipose tissue is metabolically active, although some depots appear to serve only a mechanical function and may be metabolically inactive (1). Adipose tissue is the body’s largest store of energy, accounting for 10-15 kg in a non-obese young adult. Supplying 3500 kcal per pound, 15 kg of body fat stores 135,000 kcal. Adipocytes (fat cells) regulate the energy balance of the entire organism by storing excess energy intake as triacylglycerols and releasing stored energy when demands are not met by other means. Each adipocyte contains roughly 0.04-0.06 ug of fat which accounts for ninety percent of the weight of adipose tissue (2).
Lipolysis is the process of breakdown and release of triacylglycerols (TGs) in adipocytes and is intensely regulated. Many physiological factors stimulate and inhibit the breakdown of TGs into free fatty acids (FFAs) and glycerol and their mobilization into the bloodstream to be used as fuel by other cells and tissues. Feeding, fasting, exercise and stress have pronounced and rapid effects on lipolysis via hormones and other endogenous substances (see Table 1). As well, clinical conditions such as diabetes and obesity are associated with alterations in lipolysis. Age and gender are also of importance.
Table 1. Some physiological modulators of lipolysis in humans.
State | Effect on lipolysis | Regulating hormones |
Fed | Inhibits | Insulin by activity on HSL |
Fasted | Increases | Increase in catecholamines and decrease in insulin |
Exercise | Increases | Increase in catecholamines; decrease in insulin |
Age | Decreases | Reduction in SNS activity and catecholamines |
Gender | Varies | Sex hormones; regional deposit variations |
Insulin and the catecholamines are the main regulatory hormones of lipid mobilization. Insulin is the major antilipolytic hormone because of its effects on enzymes within the adipocyte. Insulin also enables the entry of glucose into the cells by inducing glucose transporter activity. Glucose serves as the backbone for the glycerol molecule to which fatty acids attach and form TGs. The catecholamines serve a dual function of stimulation and inhibition of lipolysis. This nature of this duality will be discussed further so that the reader may understand how lipolysis is tightly regulated.
Hormones such as prostaglandins, adenosine, growth hormone and cortisol have permissive regulatory effects. Endocrine effects are due to circulating hormones, such as testosterone. Others are paracrine and autocrine effects from hormones secreted by the cell itself or from neighboring cells (18, . These mechanisms will not be discussed in depth here except for their role in mediating catecholamine-induced lipolysis.
We must first become acquainted with fat cell biology to comprehend the regulation of fat loss. Lipogenesis and lipolysis can be considered the Yin and Yang of adipose tissue metabolism. Lipogenesis is the process of fat accumulation and lipolysis is that of fat breakdown and release into the bloodstream. Bryan Haycock described the various systems in his article which readers are referred to for an in depth explanation. To avoid redundancy, the systems will be summarized here but with a greater examination of the adrenergic control via the adrenoceptors.
Triacylglycerol Storage
When carbohydrates are ingested, plasma glucose levels rise causing a release of insulin from the pancreas. Circulating insulin activates enzymes (acetyl-CoA carboxylase and fatty acid synthase) by inducing phosphorylation. This catalyzes the formation of fatty acids from glucose. Fatty acid uptake by the adipocytes results from activity of lipoprotein lipase (LPL). In addition to other hormones, insulin increases synthesis and activity of LPL; catecholamines, growth hormone and testosterone inhibit LPL. Differences in LPL activity partially account for regulation of adipose tissue distribution in various deposits. This may partly explain the typical diversity in adipose tissue deposition in regional deposits and between the two genders (e.g. upper body versus lower body, visceral versus subcutaneous deposits).
As mentioned previously, insulin enables entry of glucose into the cells via induction of glucose transporter activity. Glucose is then metabolized to glycerol where, along with fatty acids in the cell, they serve as substrates for TGs and are stored. Among other lipogenic effects, insulin stimulates the esterification process within the adipocyte, where three fatty acid chains attach to a glycerol molecule to form a TG. The primary action of insulin involves dephosphorylation of hormone sensitive lipase (HSL), which deactivates the enzyme. As will be described shortly, HSL catalyses the rate-limiting reaction in TG breakdown. Consequently, insulin is frequently referred to as the anti-lipolytic hormone.
Adrenoceptors and Lipolysis
Lipolysis is the process where TG molecules are hydrolyzed to fatty acids and glycerol. These products are then moved out of the fat cell by passive diffusion, carried across the cell membrane by protein transporters, or, in the case of FFAs, re-esterified back into TG within the fat cell or others nearby. The products are circulated through the bloodstream to various tissue and organs to serve as a source of fuel. The entire process is tightly regulated by numerous factors of which only the key players will be addressed here.
Recall that the rate-limiting enzyme for lipolysis within the adipocyte is HSL. Likewise, insulin and the catecholamines regulate HSL activity. Activation of HSL is regulated by a series of metabolic processes in the cell mediated by hormones and receptors in the cell membranes. Hormones secreted by glands or neurons, circulate and bind to receptors. This hormone/receptor complex initiates events that begin in the cell membrane, progress to the cell interior and end in a physiological response that may be inhibitory or stimulatory depending on receptor type and subtype. This is commonly called the second messenger system. The hormone itself is the first messenger which stimulates production of a second messenger that acts on systems within the cell to produce a cascade of events leading to the effect of the hormone. The series of steps and signals that take place linking the receptor and the effects within the cell is called signal transduction. As in most biological systems, negative feedback may inhibit the events of this interaction. To fully understand the complexity of this system, we will look at the cascade beginning with the receptors.
The physiological response by endogenous compounds or pharmaceuticals relies on their interaction with receptors in or on a cell. As we will see in the second installment, catecholamines and drugs (ligands) act upon these receptors and regulate metabolism in adipocytes and other cells. Some drugs, such as yohimbine, distinctly target a specific receptor to mediate a desired (or undesired) response. Let us examine what transpires when a ligand binds with a receptor.
Receptors are classified based on their structure and mechanism of action. The two classes of receptors are those that reside in the cell (intracellular receptors, such as steroid receptors) and those that span the cellular membrane and transfer an extracellular signal to an intracellular response. The G-protein-coupled (guanine nucleotide regulatory proteins) receptors are a large family of cell-surface receptors. The major receptors in the adipocyte membrane are the adrenergic receptors, or adrenoceptors, and are members of the G-protein family. Their most common feature is the long polypeptide chain that loops back and forth through the membrane seven times. This chain is physically and functionally linked to G proteins within the cell.
The catecholamines norepinephrine and epinephrine serve as the first messengers by binding to the adrenoceptors and stimulating the cascade system described above. The signal transduction depends on the type of adrenoceptor of which there are two types: alpha- and beta-adrenoceptors. These are further subtyped depending on structure, pharmacological response and second messenger. The alpha2– and beta-adrenoceptors share the same second messenger: cyclic adenosine monophosphate (cAMP). The alpha1-adrenoceptors’ second messenger is calcium or phosphatidylinostiol and has less significance in lipolysis.
The heterogeneity of the adrenoceptors on the adipocyte offers a dual control of lipolysis by differential recruitment by the catecholamines. This is based on their relative affinity for the different subtypes. Lipolysis is mediated primarily by three beta-adrenoceptors: beta-1, beta-2 and beta-3 (b 1, b 2, and b 3, respectively). Stimulation of the alpha2-adrenoceptor (a 2-adrenoceptor) is anti-lipolytic. That is, its activation inhibits lipolysis within the cell. To understand this dual regulation, let us take a look at the cascade mechanisms activated by surface cellular adrenoceptors.
G-coupled protein receptors, such as the adrenoceptors, link with G proteins just inside the cell membrane. The G proteins consist of three subunits with different binding and characteristic effects. This is commonly called the G protein effector system. When either of the catecholamines bind to an adrenoceptor on the adipocyte, it causes a conformational shift that activates one or more of these G protein subunits. Specificity of the hormonal response is regulated by one of the subunits coupling to different effector molecules within the cell. This specific coupling activates a cascade of signals within the cell ultimately leading to a physiological response.
The two families of the G-proteins that are involved with control of lipolysis are Gs and Gi. Beta-adrenoceptors couple with the Gs form and thereby activate adenylyl cyclase (AC), the key enzyme which produces cAMP. HSL, the enzyme that catalyzes breakdown of TGs, is regulated by cAMP. Therefore, activation of AC initiates metabolism of TGs.
As stimulation of the beta-adrenoceptors can be thought of as the “on” switch for lipolysis, the a 2-adrenoceptor can be considered the “off” switch. a 2-Adrenoceptors have been less studied than the beta-adrenoceptors; however, they are known to be linked to the Gi protein subunit complex. Activation of the a 2-adrenoceptor attenuates the production of cAMP by its inhibition on AC. The exact mechanism of this inhibition is not clearly understood. Several theories have been presented; the currently accepted is the dissociation of the subunits of the proteins from the Gi-protein complex, thereby inhibiting further transduction of the signal to AC (3, 4).
Recall that TGs are the lipid forms of energy stored in adipose tissue. Catecholamine-adrenoceptor mediated stimulation of AC promotes elevation of cAMP and, in turn, increases activity of cAMP-dependent protein kinase A (PKA). PKA activates HSL by phosphorylation and its translocation to the lipid droplets of TGs. Activated HSL breaks down TG to diacylglycerol (DG) and monoacylglycerol. Monoacylglycerol lipase (not under hormonal control) then hydrolyzes DGs to free fatty acids and glycerol. The net products of the breakdown of one TG are three molecules of fatty acids and one of glycerol.
Glycerol passively diffuses through the cell membrane into the extracellular fluid and bloodstream. The free fatty acids may have several fates. A portion of the liberated FFAs remains in the adipocyte to be re-esterified back to TG within the cell. The remaining are carried across the membrane by a transport protein (5) into the extracellular fluid and pass into the bloodstream, or they can be taken up by surrounding adipocytes and re-esterified into TGs. The fate of free fatty acids after TG breakdown are determined by a number of factors which will be discussed in detail in Part II.
Lipolysis is largely controlled by the amount of cAMP within the cell. Other hormones affect lipolysis at the post-receptor level by acting on specific enzymes or cofactors to increase cAMP or inhibit re-esterification of the FFAs. They will not be discussed in this article except where necessary.
Interplay of b – and a 2-adrenoceptors
An examination of the role of adrenoceptor affinity for the catecholamines will elucidate the beta/a 2-adrenoceptor interplay in regulation of lipolysis. Recall that the adrenoceptor population on adipocytes is heterogeneous. That is, there is a mixture of a 2–adrenoceptors and the subtypes of beta-adrenoceptors; the number and density of each varies among deposits of adipose tissue. The relative proportions of each greatly determine the biology of the adipose tissue. Brown adipose tissue (BAT), which is the major site of thermogenesis, which maintains body temperature, has a higher density of b 3-adrenoceptors than the other beta-adrenoceptors. The b 1– and b 2-adrenoceptors undergo desensitization and downregulation quickly, whereas the b 3-adrenoceptors do not. Although the metabolism and role of BAT is still controversial, it has been proposed that the b 3-adrenoceptors are essential for continuation of catecholamine responses under increased or sustained sympathetic activity (6).
The heterogeneity of adrenoceptors varies among species, gender, and deposits of adipose tissue. This topic in itself could easily constitute another article, but will be summarized here. Studies indicate that, of the species examined, human adipose tissue contains the highest density of a 2-adrenoceptors (7). Men and women display regional differences in lipolysis mostly due to varying populations of the adrenoceptors. Non-obese women generally have more subcutaneous fat in the gluteofemoral area (buttocks and thighs) than other areas. Non-obese men generally have a uniform distribution of subcutaneous fat. Obesity, however, exhibits pronounced gender differences. Obese women tend to accumulate fat in the gluteofemoral and lower abdominal areas. This is commonly called a gynoid pattern of fat distribution. Obese men typically accumulate fat in the subcutaneous abdominal area, which is called an android pattern. The exact causes of these gender differences in regional adiposity are neither absolute nor fully understood.
Hormones such as testosterone and estrogens are known to affect adrenoceptor gene expression. Studies with other species demonstrate that androgen administration increased a 2-adrenoceptor expression in intra-abdominal adipose tissue in hamsters. The same up-regulation of a 2-adrenoceptors has not been yet demonstrated in human males (8, 18). Conversely, in studies with humans testosterone administration up-regulated beta-adrenoceptors, especially in the abdominal region (9). Estrogen may have a role in the paracrine control of adipocytes, although the exact mechanisms are not clearly understood (10, 11).
Body fat also differs among regional deposits due to differences in metabolic activity. Subcutaneous body fat comprises about 80% of all adipose tissue (12). Visceral adipose tissue surrounds the stomach and the intestines, is drained by the portal vein and has direct access to the liver. Activity is highest in the visceral region where more fat is mobilized during times when there is a need for rapid energy supply, such as during physical exercise or lactation. Metabolic activity is lowest in the gluteofemoral area followed by the abdominal subcutaneous deposits. Catecholamines are most active in the visceral area, followed by the subcutaneous abdominal and gluteofemoral areas. These regional variations are partially due to differences in adrenoceptor populations as well as blood supply (13, 14).
In vitro and in vivo studies show that adrenoceptor interplay accounts for the potency of catecholamine action and may be partly responsible for gender and site differences in lipolysis (13, 5, 14). Beta-adrenoceptors follow the order of expression: visceral>abdominal subcutaneous>peripheral subcutaneous, which includes gluteofemoral adipose tissue. a 2-Adrenoceptors follow the opposite order. Indeed, women generally have larger deposits of adipose tissue in the buttocks and thighs because of a higher number of a 2-adrenoceptors and reduced number of b 1– and b 2-adrenoceptors. Later discussion will elucidate how these site-specific differences in adrenoceptors affect regional fat mobilization.
The main factor associated with the preponderance of a 2-adrenoceptors is the increase in fat cell size (15, 16). Studies determined that gluteofemoral adipocytes in women are larger than those in men, thereby contributing to a higher a 2-antilipolytic effect of the catecholamines in gluteofemoral sites (15). The inverse has been observed in subcutaneous abdominal adipocytes in men (14, 17). Although the association has not been confirmed, it appears that this may be governed by sex hormones at the post-receptor level. Cell swelling and short-term modifications of cell volume could also affect metabolism and gene expression in the adipocytes. Therefore, fasting and cold exposure induce a reduction in fat cell size and a concomitant decrease in a 2-adrenoceptor binding sites (18).
The influence of obesity and fat deposits on lipolysis is not fully understood. However, an increase in fat cell size and cell number, such as seen in obesity and in aging, will increase the density of a 2-adrenoceptors and hinder weight loss attempts.
Regional variations in insulin receptor affinity and in post-receptor signaling contribute to site and gender variations as well (14, 19). In addition to their proposed effects on adrenoceptor expression, the sex hormones influence post-receptor mechanisms of lipolysis. Testosterone inhibits LPL although it is not known if this is solely adrenoceptor mediated. Estrogens and progesterone stimulate LPL and preferentially affect the gluteofemoral adipocytes.
In vitro and in situ studies have demonstrated that a 2-adrenoceptors may be highly significant in their regulatory role in lipolysis. Advanced techniques and pharmacological approaches using selective a 2– and b -agonists and antagonists reveal gender and tissue specific differences in adrenergic control of fat cell biology (14, 20-23). The next installment will discuss various interactions of a 2-adrenoceptors: the SNS, local blood flow, and relative affinity of the catecholamines for adrenoceptors in adipose tissue. Following this, we will consider how a 2-antagonists may fit into the pharmacological approach to fat loss.

References:
Garg A, Fleckenstein JL, Peshock RM, Grundy SM. Peculiar distribution of adipose tissue in patients with congenital generalized lipodystrophy. J Clin Endocrinol Metab 1992; 75:358-361.
Ryan TJ, and Curri SB. The structure of fat. Cl Dermatol 1989; 7:37-47.
Smith CA and Wood EJ. Cell Biology. 1994. Chapman and Hall
Limbard LE, MacMillan LB, Keefer JR. Specificity in a2-adrenoceptor signal transduction: receptor subtypes coupling to distinct signal transduction pathways and localization to discrete subdomains in target cells. p. 139-145. In: Adrenoceptors: Structure, Function, and Pharmacology, Ruffolo, RR ed. 1995. Harwood Academic Publishers.
Arner P. Regulation of lipolysis in fat cells. Diab Rev 1996; 4:450-463.
Langin D, Tavernier G, Lafontan M. Regulation of beta 3-adrenceptor expression in white fat cells. Fundam Clin Pharmacol 1995; 9:97.
Castan I, Valet P, Quideau N, et al. Antilipolytic effects of a 2-adrenergic agonists, neuropeptide Y, adenosine, and PGE1 in mammal adipocytes. Am J Physiol 1994; 266:R1141.
Dieudonne MN, Pecquery R, Leneveu MC, et al. In vitro up-regulatory effect of testosterone on a 2-adrenoceptor expression in adipose tissue. Endocrine 1994; 2:567-570.
Rebuffe’-Scrive M, Marin P, Bjorntorp P. Male adipose tissue. Int J Obes 1989; 13(supp 1):181.
Price TM, O’Brian SN. Determination of estrogen receptor messenger ribonucleic acid (mRNA) and cytochrome P450 aromatase mRNA levels in adipocytes and adipose stromal cells by competitive polymerase chain reaction amplification. J Clin Endocrinol Metab 1993; 77:1041-1045.
Bjorntorp P. 1997. Hormonal control of regional fat distribution. Hum Reprod 1997; 12 (suppl 1):21-25.
Arner P. Obesity and the adipocyte: regional adipocity in man. J Endocrin 1997; 155:191-192.
Ramsey TG. Fat cells. Endocrin Metab Cl N Am 1996; 25:847-870.
Bouchard C, Despres JP, Mauriege P. Genetic and nongenetic determinants of regional fat distribution. Endocrin Rev 1993; 14:72-93.
Richelson B. Increased alpha2– but similar beta-adrenergic receptor activities in subcutaneous gluteal adipocytes from females compared with males. Eur J Clin Invest 1986; 16:302-309.
Wahrenberg H, Lonnqvist F, Arner P. Mechanisms under-lying regional differences in lipolysis in human adipose tissue. J Clin Invest 1989; 84:458-467.
Leibel RH, J Hirsh. 1987. Site and sex-related differences in adrenoceptor status of human adipose tissue. J Clin Endrorinol Metab 1987; 64(6):1205-1210.
Lafontan M, Berlan M. 1995. Fat cell alpha2-adrenoceptors: the regulation of fat cell function and lipolysis. Endocr Rev 1995; 16(6):716-738.
Maurige P, et al. Regional difference in adipose tissue lipolysis from lean and obese women: existence of postreceptor alterations. Am J Physiol 1995; 269(2 pt 1): E341-E350.
Bjorntorp P. The regulation of adipose tissue in humans. Int J Obesity 1996; 20:291-202.
Arner P, Kriegholm E, Engfeldt P, Bolinder J. Adrenergic regulation of lipolysis at rest and during exercise. J Clin Invest 1990; 85:893-898.
Millet L, Barbe P, Lafontan P, Berlan M, Galitzky J. Catecholamine effects on lipolysis and blood flow in human abdominal and femoral adipose tissue. J Appl Phys 1998; 85:181-188.
Lafontan M, Betuing S, et al. Regulation of fat-cell function by a 2-adrenergic receptors. Adv Pharmacol 1998; 42:496-498.
About the author
Elzi has spent the last several decades trying to determine where 'home' is: from New York, Maine, California, Oregon and now Texas. As well, her career has encompassed tool & die apprentice, forest ranger, assistant extension agent, mother, sheep and horse rancher, and mad research scientist. She has also been a competitive bodybuilder, but has found true joy in powerlifting. When Elzi is not playing fetch with her 1200 lb four-footed buddy, she is most happy in the gym and in a research lab.
Leave a Reply
You must be logged in to post a comment.