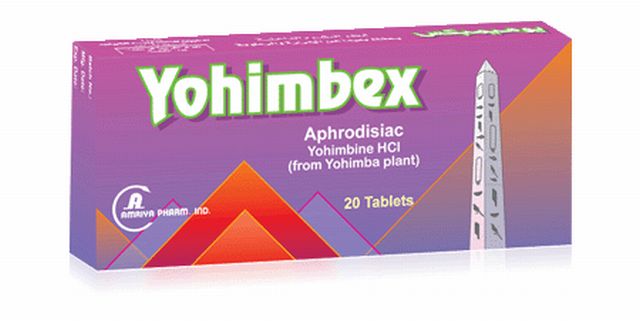
In the previous installment of this article, we discovered the regulatory role of adrenoceptors on lipolysis by action of the catecholamines. This installment examines the relevance of the sympathetic nervous system in mediating levels of catecholamines in the body and the interactions of this system with adipose tissue. The role of a 2-adrenoceptors and a pharmacological approach that mediates these receptors is also discussed.
The Sympathetic Nervous System and a 2-Adrenoceptors
It is widely accepted that lipolysis is modulated by the sympathetic nervous system (SNS) and possibly the parasympathetic nervous system (PNS). The SNS and the PNS are the two arms of the autonomic nervous system of the body. The SNS, often called the “fight-or-flight” system, is a network of motor neurons that innervates smooth muscle, cardiac muscle and glands. The SNS mobilizes the body during extreme situations such as stress and exercise. The PNS, sometimes called the “resting and digesting” system, serves to counterbalance the effects of the SNS and conserve energy. The SNS may stimulate a gland to secrete or smooth muscle to contract, whereas the PNS inhibits that action. Generally, the SNS and PNS innervate the same organs; although, the SNS innervates more organs than the PNS. While adipose tissue is innervated solely by the SNS, the PNS may indirectly influence lipolysis.
Both systems comprise of neurons, and each neuron ends in a terminal synapse. These synapses mediate the transfer of information from one neuron to another or to an effector (target) cell. That information may be in the form of electrical impulses (flow of ions) or chemicals. Certain signals are transmitted while others are blocked. Just inside the terminal are vesicles containing neurotransmitters, the chemical signals. In response to a nerve impulse the neurotransmitters are released from the vesicles into the synaptic cleft, a narrow space between the presynaptic terminal and the postsynaptic membrane of a nerve or an effector cell. Neurotransmitters diffuse across the synaptic cleft and bind to specific receptors on the effector cell. They may also diffuse into the bloodstream or be degraded by enzymes. Some neurotransmitters are taken back up into the presynaptic neuron to be recycled in a process called re-uptake.
The major neurotransmitter of the PNS is acetylcholine (ACh), which binds to nicotinic and muscarine receptors. The two neurotransmitters of the SNS are acetylcholine and norepinephrine (NE). ACh is degraded quickly by acetylcholinesterase; hence its effects are short-lived. Stimulation of the SNS increases release of neurotransmitters inducing a response in the effector cells, which may be excitatory or inhibitory depending on the nature of the receptor that binds the neurotransmitter. Thus, the SNS regulates energy intake and expenditure according to genetic and environmental influence.
SNS stimulation increases plasma levels of the catecholamines by inducing secretion of NE from postganglionic terminals and release of epinephrine (E) from the adrenal medulla. Although NE lingers in the synaptic cleft for a longer period of time than ACh, NE has several fates. A portion of the NE diffuses out of the synaptic cleft into the bloodstream. Enzymes such as monoamine oxidase and catechol-O-methyltransferase (COMT) degrade a portion of NE. Much of the neurotransmitter is actively transported back into the terminal that released it and recycled. This re-uptake is moderated by a -adrenoceptors.
a 2-Adrenoceptors are found in the cell membrane of the neuron axon terminals and mediate rate neurotransmitter release. Some evidence shows that a 1-adrenoreceptors may be present on presynaptic membranes as well, but their existence is still disputed (1). When NE is released from the vesicles of the terminals, they come into contact with and stimulate the a 2-adrenoceptors, inhibiting further release of these same neurotransmitters. Such is the feedback system for NE release in the SNS.
Many pharmaceuticals interact with presynaptic a -adrenoceptors interfering with NE-mediated regulation of NE release and re-uptake. a 2-Antagonists are compounds that block the inhibiting effect of the a 2-adrenoceptors and, therefore, interfere with re-uptake of the synaptic NE. This allows NE to linger longer in synaptic clefts producing excessive stimulation and diffusion of excess NE into the bloodstream. a 2-Antagonists reserpine and yohimbine enhance NE release and inhibit NE re-uptake.
a 2-Adrenoceptors are present on many tissues and mediate a variety of functions. Pre- and postsynaptic a 2-adrenoceptors found on central and peripheral neural terminals mediate noradrenergic, cholinergic and serotonergic receptors. Recall that activation of these a 2-adrenoceptors inhibits release of neurotransmitters. Thus, blockage of the adrenoceptors will increase neurotransmitter release. As well, a 2-adrenoceptors are located on many other tissues and have important pharmacological implications. Some of these are discussed further when we address use of pharmaceuticals targeting the a 2-adrenoceptors for fat loss and their possible side effects.
a 2-Adrenoceptor Subtypes
Receptor-binding studies have demonstrated that several subtypes exist for alpha-adrenoceptors types, depending on species and tissue (2,3). In addition to genetic coding, pharmacological response to agonists and antagonists determine the classifications depending on their binding potency to the receptors. The most common probes used in these studies are agonists, such as clonidine, and antagonists: yohimbine and yohimbine-like compounds such as rauwolscine, corynanthine. Each of these compounds has various binding affinities for the alpha-adrenoceptors types. Some, such as yohimbine, exhibit weak binding to a 1-adrenoceptors as well as high affinity for the a 2-adrenoceptors.
As mentioned previously, species and tissue differences exist in a 2-adrenoceptor subtypes. For instance, human brain cortex presynaptic a 2-adrenoceptors are classified as a 2A or a 2D (4). Primarily a 2A-adrenoceptors mediate vascular effects, such as changes in blood pressure (5). Whereas, human kidney presynaptic a 2-adrenoceptors are classified as a 2C (6), further binding studies established that human adipocytes express only the a 2A-adrenoceptor subtype (7, 8).
These a 2-adrenoceptor subtypes are differentially regulated by their affinity for the physiological catecholamines and sensitivity to downregulation. The functional roles for the a 2-adrenoceptors continue to be explored. Pharmaceutical agonists and antagonists are being developed with greater selectivity for the individual subtypes. They may be used as therapeutics for treating glaucoma, hypertension, non-insulin dependent diabetes and as adjuncts to general anesthesia.
The SNS, a 2-Adrenoceptors and the Catecholamines
Catecholamines stimulate the adipocyte adrenoceptors on the basis of their relative affinity for each type of adrenoceptor. These hormones preferentially recruit the a 2-adrenoceptor at lower catecholamine levels than the beta-adrenoceptors, especially in tissues where a 2-adrenoceptors predominate (9,10). Consequently, the a 2-adrenoceptors will be recruited before the beta-adrenoceptors.
One study demonstrates that a 2-adrenoceptors modulate lipolysis at rest, whereas lipolysis is modulated by the beta-adrenoceptors during exercise (9). During physical activity, increased levels of E in the extracellular fluid maximally stimulate the beta-adrenoceptors and mask the inhibitory effect of the a 2-adrenoceptors. a 2-Adrenoceptors may exert a permanent inhibition on lipolysis, contributing to a tonic inhibitory component influenced by catecholamines on ‘basal lipolysis’ (11). In vitro studies support this observation by demonstration that many G proteins have a significant level of basal activity in the absence of an agonist (12). Therefore, the a 2-adrenoceptors could be considered the major lipolysis-regulating adrenoceptor on adipocytes.
To illustrate the regulatory mechanism of the dual adrenoceptors on the fat cells, let us consider the a 2-adrenoceptor as a ‘brake’ on lipolysis in the cell. At rest the a 2-adrenoceptors apply a slight pressure to the brakes on lipolysis even in the absence of an agonist, a compound which binds and stimulates the receptor. A slight rise in extracellular norepinephrine, such as seen in mild SNS stimulation (sitting at the computer typing all day), will increase the number of a 2-adrenoceptors being activated with a small number of beta-receptors activated as well. Since the a 2-adrenoceptors outnumber the beta-receptors in humans, this will apply the breaks to lipolysis harder, decreasing-lipolysis. During exercise, concentrations of NE and E from the SNS and the adrenal gland increase in the blood and fat tissue extracellular fluid. These higher levels of catecholamines increase stimulation of the beta-adrenoceptors, which then overshadow the a 2-adrenoceptor-induced activity. Basically, stimulated beta-adrenoceptors cut the brake cable on lipolysis and start the train of events promoting and increasing fat breakdown in fat cells.
Adipocytes are only one of the many tissues within the body that have adrenergic receptors. Thus, response to activation of the SNS will vary between tissues depending on the adrenoceptor types present on the cells, the relative proportion and the second messenger system within the cell. For instance, skeletal muscle blood vessel wall cells have both a 1– and b 2-adrenoceptors with the a 1-adrenoceptors lying close to the sympathetic nerve terminals. The b 2-adrenoceptors are on the endothelial surface of the blood vessels. Therefore, SNS activation usually produces predominantly vasoconstriction mediated by the a 1-adrenoceptors, whereas an increase in E activates the b 2-adrenoceptors causing vasodilation. Another important organ with dual adrenergic regulation is the pancreas. This interaction is explained when we examine a pharmaceutical approach that blocks the a 2–adrenoceptors.
a 2-Adrenoceptors and Blood Flow
SNS activity has an additional catecholamine-mediated effect on lipolysis in adipose tissue: blood flow. A coordination of local blood flow and metabolism carries away by-products of lipolysis and supplies energy substrates to tissues and organs in times of increased demand. Changes in blood flow can facilitate or inhibit movement of substrates, such as glycerol and non-esterified fatty acids (NEFA), that arise from lipolysis. Consequently, stimulation of the SNS increases lipolysis and blood flow; low SNS activity (such as during rest) inhibits lipolysis and blood flow. However, the increase in blood flow is not in proportion to rising concentrations of NEFA and glycerol. During strenuous exercise, adipose tissue blood flow does not increase sufficiently to remove all NEFAs released by lipolysis (13). A feedback system probably exists; however, it is not well understood. Vascular adrenoceptors that affect vasoconstriction and vasodilation may be responsible for this feedback. Studies using microdialysis have shown that the interplay of a 2– and b 2-adrenoceptors mediate vascular blood flow in adipose tissue (14,15).
Up until several years ago, in vitro and in vivo investigations on plasma circulating metabolites limited measurements of metabolism in adipose tissue. A technique called microdialysis allows for local manipulation and in situ studies of adipose tissue (16,17). It can be applied to individual subcutaneous deposits enabling investigation of specific-site metabolism. Microdialysis allows infusing adrenergic-active agents, such as beta- and alpha-agonists or antagonists, to manipulate adrenergic control and monitoring adipose tissue metabolites and local blood flow. Recent studies elucidate further the role of the catecholamines on regulation of adipose tissue metabolism, especially pertaining to regional and gender differences.
E and NE stimulate blood flow in adipose tissue by activation of the b -adrenoceptors on the walls of the blood vessels causing vasodilation. Stimulation of the a 1– and a 2-adrenoceptors, also on blood vessel walls, promote vasoconstriction. The distribution of these two types of alpha-adrenoceptors and their subtypes within the blood vessel walls mediate their sensitivity to vascular controls. Some vascular beds, such as the renal bed, respond primarily to a 1-adrenoceptor modulation (5). Whereas other beds, such as in cutaneous circulation, respond primarily to a 2-adrenoceptors (19,18). SNS regulation of local blood flow in the various adipose deposits may have important implications for lipolysis (20).
Results from in vitro and in vivo studies have had contradicting results depending on techniques utilized and physiological status of subjects. Without question, in vitro and in vivo results show that a 2-adrenoceptors predominate in the femoral vascular bed (in the lower body) by using various antagonists for the specific alpha-adrenoceptors (21-23). Microdialysis studies confirm a higher concentration of glycerol in gluteofemoral than abdominal adipose deposits possibly due to reduced local blood flow in gluteofemoral sites during resting basal conditions despite lower basal lipolysis rates (14,20).
Experiments using microdialysis have shown that perfusing adipose tissue with clonidine, an a 2-agonist, promoted an increase in extracellular glycerol concentrations. Vasoconstriction by stimulation of vascular a 2-adrenoceptors may be the primary determinant in glycerol and NEFA mobilization (20,24). Vasodilating agents infused via microdialysis produced a decrease in the removal of glycerol from the extracellular space of adipose tissue (24). The resulting decrease in blood flow may reduce the net removal of lipolysis metabolites from the extracellular fluid of adipose tissue.
NEFAs produced by lipolysis within the adipocytes may be more sensitive to change in blood flow. Glycerol is water-soluble and diffuses out of the adipocytes to move freely within extracellular fluid. NEFAs are not water-soluble and must be bound to protein carriers to move out of adipocytes and into the intracellular space. Newly NEFAs will therefore linger in the interstitial space surrounding adipocytes and possibly be reutilized (re-esterified) by surrounding adipocytes. Indeed, it has been shown that reduced blood flow in adipose tissue delayed NEFA and glycerol mobilization (25,26). Therefore, vasodilation induced by an antagonist that specifically blocks the a 2-adrenoceptors in the blood vessels of adipose tissue could increase lipolysis metabolite mobilization.
In vitro and in vivo studies suggest obesity may modify the response to the catecholamines due to differences in fat cell size, adrenoceptor populations and circulation (27-31). Continued investigation is needed to explain controversies in changes of regulatory mechanisms seen in altered physiological states. Additionally, physical exercise modifies changes in adipose tissue response to SNS. Gender differences are apparent during exercise, such as higher glycerol levels in circulating blood supply and in adipose tissue of women than in men. Microdialysis investigations report higher lipid mobilization from subcutaneous abdominal adipose tissue in women (9,32). Explanations for this gender response may be differences in adrenergic receptor population and activity. Glycerol levels in men were enhanced by a -adrenoceptor blockage. However, whether this was induced by direct blockage of adipocyte alpha-adrenoceptors or those of the vascular bed was not examined.
The Sympathetic Nervous System, a 2-Adrenoceptors and Yohimbine
Thus far, this article has addressed the basic physiology of lipolysis and the interaction of the SNS and alpha-adrenoceptors. As alluded to throughout the preceding sections, manipulation of the SNS will have direct impact on lipolysis. It is useful to remember, however, that insulin is the main regulator of lipolysis. Therefore, as previously mentioned in Part 1 of this article, lowering insulin levels will allow for optimal manipulation of the SNS. Low levels of insulin increase plasma levels of catecholamines, stimulating lipolysis and loss of body fat. The SNS can also be manipulated by pharmaceuticals and naturally occurring substances. We will discover one such approach: mediation of the a 2-adrenoceptors.
Sympathomimetic compounds mimic the action of the SNS and release NE and E in addition to possessing direct b -adrenergic properties. Examples of these compounds are amphetamines, ephedrine and its various isomers. Isomers are compounds with the same formula but different molecular structure or different spatial arrangements. These differences greatly affect the activity/potency of the compound and the responses they elicit, as we will discover shortly.
Sympatholytic compounds inhibit adrenergic nerve activity in the SNS and some have direct postsynaptic adrenoceptor-blockage activity. Physiological responses of these compounds depend on several factors: chemical structure, type and subtype of adrenergic receptors (ARs), second messenger system (including G protein complex), and tissue site. Other factors include administration route of the compound, which influences absorption and metabolism, and dosage. To present a detailed discussion of pharmacology is beyond the scope of this article; therefore, only necessary details pertaining to the ensuing discussion are included.
Considered a sympatholytic, yohimbine has been used in herbal medicine for centuries. Yohimbine is one of a large family of indole alkaloids called yohimbanes. Indole alkaloids are naturally-occurring heterocylic amines derived from botanical sources. Yohimbine is the principal alkaloid found in extracts from the bark of the Pausinystlia yohimbe tree that grows in tropical West Africa and the Congo. It is structurally similar to reserpine and can also be isolated from the roots of Rauwolfia.Typical of many alkaloids, the yohimbanes have diverse pharmacological properties.
The basic yohimbane molecular structure contains five asymmetric carbons; yohimbine is one of 32 isomers within this family. The yohimbane alkaloids include antagonists that are selective for the alpha-AR. The selectivity of the various yohimbane alkaloids depends on the stereochemical configuration of the five carbon centers. That is, the shape and position of the various components of the compound determine how they interact with the receptors and potency of their response. Not only do they have differential activity at the alpha-AR types (a 1– versus a 2), but also within the subtypes (3,15,33).
Recall from the previous discussion on the a 2-AR subtypes that differences in their affinity for agonists and antagonists mediate the cell’s response. This interaction of yohimbane compounds and the AR subtypes determine their use as pharmacological tools and therapeutic agents. Therefore, the effects of the various yohimbanes will vary, as we shall see in our examination of the use of yohimbine.
Synthesized yohimbine and its isomer rauwolscine have been used as pharmacological tools to differentiate the alpha-ARs due to their selectivity as antagonists for the a 2-AR. Another isomer, corynanthine, is used for its selectivity for the a 1-AR. They have served as probes for classification of AR types and to assess a 2 adrenergic functions in man for several decades. Herbal preparations from plant parts, however, have been used as aphrodisiacs and euphorics for centuries. Recently, yohimbine has been promoted as a dietary supplement to enhance athletic performance and fat loss. This and various clinical applications will be examined in this article.
Early investigations demonstrate the activity of yohimbine as an a 2-antagonist that increases NE release and induces a hyperadrenergic state (33). Pharmaceutical studies show that yohimbine has high selectivity for the a 2-AR and weak affinity for the a 1-receptors. Its isomer rauwolscine has higher selectivity for the a 2-AR with little or no selectivity for the a 1 type. Later tissue and cells studies explained the mechanisms of various effects of yohimbine when administered to humans and other species by revealing the presence of a 2-AR and their functions at several sites within the body. Differential affinity of yohimbine and its isomers for the a 2-AR subtypes may also explain variability in tissue-specific responses.
Clinically, yohimbine has been administered to induce anxiety in psychiatric patients, orthostatic hypotension and other autonomic failure conditions, adjunct therapy for opiate withdrawal, and male organic impotence (15,34). It is widely used by veterinarians to reverse sedation or anesthesia in animals. Other therapeutical applications currently under research are as a glucose-dispersal agent for treatment of non-insulin dependent diabetes and to treat adverse effects of anti-depressants.
Yohimbine and Fat Loss
Since a 2-ARs are present on adipose tissue and inhibit lipolysis, yohimbine has been proposed as a pharmaceutical approach to fat loss. In vitro results demonstrated that E, a non-selective agonist for a /b -ARs, produced less lipolysis than a selective beta-agonist (33). However, when an a 2-antagonist, such as yohimbine, was added, E induced the same rate of lipolysis as the beta-agonist. Clinical investigations report yohimbine administration (0.2 mg/kg total body weight) increases plasma levels of NEFAs and glycerol in obese and non-obese women as well as in men (26,35,36). Yohimbine increased weight loss when used with hypocaloric diets in several studies by preventing an adaptive lowering of the SNS that usually occurs with most calorie-restriction (37). Resting energy expenditure did not change in a group of obese and lean women when administered yohimbine (38). However, exercise energy expenditure in same subjects was significantly potentiated along with accompanying parameter of lipolysis
Results from many studies conclude that the primary lipid-mobilizing effect of yohimbine is stimulation of the SNS (26,34,35). A beta-antagonist was administered to non-obese and obese subjects 60 minutes before yohimbine. This suppressed the b -AR effect of lipolysis induced by increases in NE levels. Plasma NEFA levels decreased, but plasma levels of NE were unchanged (34). However, yohimbine still had a transient lipid mobilizing effect evidenced by increased levels of NEFA after b -AR blockage (34,35). These results indicate that only a minor part of the lipid-mobilizing effect of yohimbine is attributed to direct blockage of the lipolysis inhibiting a 2-ARs on adipose tissue.
Discrepancies exist in methodology used in yohimbine and weight loss research. Considering that increases in NE levels after oral yohimbine is dose dependant (39), most studies have shown optimal dosage to be 0.2 mg/kg (8). Some studies have used much smaller dosages with less significant results. Another cause of discrepancy is timing of yohimbine administration. The lipid-mobilizing effects of yohimbine are completely negated when administered with or after a meal (35). Administration of a 2-antagonists such as yohimbine increases insulin secretion during glucose stimulation. This is attributable to the blockage of the a 2-ARs on pancreatic beta-cells and to concomitant stimulation of the pancreatic beta-ARs. Yohimbine was administered to test subjects in earlier studies which caused increased insulin secretion to blunt its lipid mobilizing effects (40). However, in fasting conditions, yohimbine does not increase insulin levels. These results demonstrate the importance of nutritional status when administering a 2-antagonists as lipid-mobilizing agents.
Studies suggest yohimbine may increase vasodilation in adipose tissue. Subjects administered yohimbine showed significantly increased levels of plasma NEFA when they changed from a supine position to an upright position (26). The increase in NEFA levels observed were probably due to b -AR stimulation of lipolysis and possibly an increase in net outflow of NEFA from adipose tissue due to yohimbine-induced decrease in local vasoconstriction. When nitroprusside, a vasodilator, was infused by microdialysis, extracellular glycerol concentrations decreased and escape of ethanol increased in adipose tissue (20,24). This suggests that the escape of glycerol from the extracellular spaces in adipose tissue can be accelerated by increased blood flow. The peripheral vasodilation effects of many a a-antagonists are known. However, the direct vasodilation effects in adipose tissue by yohimbine will need to be conclusively assessed by techniques such as microdialysis.
Increased peripheral vasodilation by blockage of the blood vessel wall a 2-ARs may increase systemic distribution of lipolysis by-products. Submaximal-intensity exercise could enhance net lipolysis in combination with yohimbine administration. Microdialysis studies suggest that NEFAs accumulate within adipose tissue during strenuous exercise due to limited transport into systemic blood supply (13). Recall that exercise-induced increases in blood flow does not rise in proportion to NEFA production (41,42). Therefore, the rate of systemic NEFA delivery does not increase simultaneously with increasing intensity of exercise. Accumulation of NEFA in adipose extracellular spaces may possibly exert a feedback inhibition on lipolysis. As well, vasoconstriction may be induced by high concentrations of NEFA or high sympathetic stimulation (43). Increased peripheral vasodilation in adipose tissue effected by a 2-antagonists may enhance removal of lipolysis by-products during low- to moderate-intensity exercise. Additionally, higher systemic transport of NEFA may increase their uptake into working muscles.
Considering the research that is available thus far, it is conceivable that the optimal plan for administrating yohimbine as a lipid-mobilizing agent would be early mornings after an overnight fast and several hours before breakfast. The relatively low levels of plasma glucose would not stimulate a yohimbine-potentiated increase in insulin that would otherwise negate its lipolytic effects. Utilization of body fat stores would be optimal with low insulin levels and lipid-mobilizing stimulation of the SNS. Conceivably, yohimbine administration could be followed in two to three hours by ingestion of a high-protein, high-fat meal. Protein ingestion will cause only a transient rise in plasma glucose levels with a concomitant rise in insulin secretion. Accompanying dietary fat will slow digestion and absorption of protein in the same meal. Therefore, the resulting insulin spike will only transiently blunt lipolysis.
Most of the studies with yohimbine as adjunct treatment for weight loss report very few side effects in subjects. However, whatever the therapeutic use, side effects will occur due to the blockage of a 2-ARs on other tissues. Dosage, nutritional status, and general physiological condition of the individual can modify these side effects. Side effects may consist of skin flushing, headaches, and excitement. Men may experience an increase in or prolonged erections possibly due to increase in vasodilation in the penile tissue, which has a high density of a -ARs.
Few study subjects experienced changes in blood pressure or rise in heart rate. This may be explained by blockage of both vascular a 1/a 2-ARs and minimizes the vascular responses induced by catecholamine release (15,33,44). However, vascular changes have been reported when yohimbine administration is accompanied by exercise. Anecdotally, many people dosing with yohimbine report rapid increase in heart rate during cardiovascular (aerobic) activity. It is wise to monitor heart rate closely when combining the two.
Some individuals experience profuse sweating and increased salivation at higher doses (45,46). Yohimbine will block the a 2-ARs mediating acetylcholine and effect the cholinergic system as mentioned in the beginning of this article installment. Other side effects may include water retention and increased colon excretion (47).
Most studies reported that subjects experienced little or no side effects with single oral dosages ranging from 5-20 mg. Those studies basing dosages on bodyweight (0.2mg/kg) also reported few problems with side effects. Yohimbine administration to subjects in psychiatric research induced episodes of anxiety in individuals with pre-diagnosed anxiety disorder (48). Control subjects did not experience similar effects. Central nervous system effects are dose dependent. High oral dosages (40 mg ) and intravenous administration may significantly increase plasma E levels with resulting increase in heart rate and blood pressure (49,50).
Several contraindications exist that individuals should be aware of when considering dosing with yohimbine. As with most any a 2-antagonist, yohimbine can acutely increase blood pressure in hypertensive patients. It should also not be combined with other drugs that are known to inhibit neuronal uptake or metabolism of NE, such as some anti-depressants. Individuals should also consider the tendency of yohimbine to evoke psychiatric reactions in those who are predisposed to anxiety or panic episodes (51). Yohimbine will act synergistically with ethanol to increase alcohol intoxication by increasing NE turnover albeit through different mechanisms (52).
Yohimbine Sources
In addition to other factors listed above, the magnitude and variety of responses to yohimbine depends on source. Pharmaceutical yohimbine is available by prescription in 2.4-mg tablets. Commonly called ‘yohimbe’, it is also widely available in over-the-counter herbal formulations and extracts. Anecdotal and personal observations support that responses to administration of herbal formulations and extracts are more varied and pronounced than from pharmaceutical yohimbine hydrochloride.
Recall from the earlier description of yohimbine that it is the principle alkaloid present in the bark of P. yohimbe. Of the 32 isomers of yohimbane, yohimbine, rauwolscine, and corynanthine are the most studied. While less research is available on the other isomers, several have been isolated and all have similar pharmacological effects with variation in potency and selectivity. For instance, yohimbine and rauwolscine are potent a 2-antagonists, with rauwolscine having more selectivity for the a 2-ARs and less a 1-AR activity than yohimbine, which also has weak a 1-antagonist activity. Conversely, corynanthine and raubasine are selective a 1-antagonists. They also appear to have differential affinity for the various a 2/a 1-ARs (3,15,33).
The herbal formulations probably contain many of the other yohimbane alkaloids beside yohimbine. One recent investigation reported the presence of many alkaloids in 26 over-the-counter yohimbe products including dry bark and liquid extracts (53). Extraction, separation and analysis showed that authentic yohimbe bark contained 7089 ppm (0.7%) yohimbine and 3.9% total alkaloids. Most products analyzed contained very little (<0.1-0.5 ppm) yohimbine. Some of the products contained other alkaloids in addition to yohimbine. Liquid extracts contained the highest concentration of alkaloids, including yohimbine. None of the commercial products furnish information on the extraction process, and many provide no information on standardization of their product for yohimbine content. This author contacted a well-known supplement company to determine the extraction and standardization process for their herbal yohimbe product. Their reply was no more informative than what is stated on the product label and they were non-responsive to further inquiries.
Many people dosing with the herbal preparations report increased intensity of undesirable side effects. Considering that these preparations may have other yohimbane alkaloids present as well as yohimbine, various physiological effects may result from activity on both a 1– and a 2-ARs, and possibly from blockage of 5-HT and dopamine receptors (33). Also, central nervous system (CNS) activity may be increased. Individuals dosing with the herbal products should consider starting with a low dosage and raise it only as their tolerance to side effects increases.
The great variability in response to yohimbine amongst individuals drove researchers to investigate the drug’s bioavailability and pharmacokinetics. Yohimbine is rabidly absorbed and eliminated from plasma (half-life is ~0.6 hr). However, it’s apparent long duration of pharmacodynamic effects does not equate with its short plasma life. Plasma levels of yohimbine were found to be greater in obese women subjects compared to lean counterparts possibly due to decreased clearance and volume of distribution (34). Some drugs bind to plasma proteins, the concentration of which some are increased in obesity (54). Data from intravenous (IV) and orally administered yohimbine show different pharmacokinetic profiles in test subjects (55,56). Although yohimbine is rapidly eliminated in both routes of administration, the clearance rate for IV yohimbine is much lower.
Although oral yohimbine is quickly absorbed, bioavailability is highly variable, ranging from 7% to 87% (mean was 30%) (55). Clearance is also variable, with only a small proportion of yohimbine eliminated unchanged in the urine (57). Chronic administration of yohimbine may lead to extensive tissue distribution and increased concentrations in effector compartments. Animal studies and the observation of a 3-4 week latent period before improvement in erectile performance in treatment of impotence with yohimbine (57,56) supports a longer term elimination phase. These findings indicate the appearance of active metabolites with longer half-lives.
Two metabolites of yohimbine have been detected in human plasma (58). One of these metabolites, 11-hydroxyyohimbine (11-OHY), is the dominant metabolite with a longer half-life (6.0 hr) than the parent compound. Binding assays demonstrate the affinity of 11-OHY for a 2-ARs with slightly less potency than yohimbine for adipocyte a 2-ARs (34). However, its potency is well within the range found for various a 2-antagonists to induce various pharmacological effects.
Characteristic of other highly lipid-soluble drugs, yohimbine quickly crosses the blood brain barrier inducing central nervous system effects in many subjects (56,59). The level measured in rat brain tissue increases linearly with dose (60). 11-OHY is less lipid-soluble and is less likely to enter the brain. Since this compound has the same efficacy as yohimbine, it may have therapeutic use as an a 2-antagonist and induce less side effects than the parent compound.
The use of a 2-antagonists may be a pharmacological approach to improve lipid mobilization and energy expenditure. Focusing on the manipulation of the adrenergic system of the fat cell and tissues offers two possible strategies. The first one is the historical use of beta-agonists, which Bryan Haycock described in his article (see Part 1 of this article). Some of these drugs suffer limitations because of limited selectivity for beta-AR subtypes, and undesirable side effects. These include stimulation of heart rate, shaky hands, tremors, insulin-secretory action, and susceptibility of downregulation in various tissues and organs.
Targeting the a 2-ARs is another strategy that is now being explored in the research. a 2-Antagonists have exhibited some success in enhancing fat mobilization. Continued research may demonstrate how this approach can be augmented with hypocaloric diet and exercise to provide an effective and safe alternative to use of beta-agonists. Continued studies should clarify the pharmacokinetics of yohimbine and its metabolites and how they interact with the various subtypes of the a 2-ARs on various tissues leading to tissue specific effects. Such information will optimize availability and half-life of these compounds during long-term use while minimizing undesirable side effects.

References:
1. Starke K, Trendelenburg AU, Limberger N. Presynaptic a 2-adrenoceptors: subtype determination. In: “Adrenoceptors: Structure, Function, and Pharmacology”, Ruffolo, RR ed. Harwood Academic Publishers. 1995:99-108.
2. Byland, DB. Subtypes of a 1– and a 2-adrenergic receptors. FASEB J 1992; 6:832-839.
3. Heible JP, Ruffolo RR, Starke K. Identification, characterization and subclassification of a 2-adrenoceptors: an overview. In: “a 2-Adrenergic Receptors: Structure, Function and Therapeutic Implications”. Lanier SM, Limbird LE eds. Harwood Academic Press. 1997:1-18.
4. Raiteri M, Bonanno G, Maura G, et al. Subclassification of release-regulating a 2-autorecptors in human breain cortex. Br J Pharmacol 1992; 107:1146-1151.
5. Piascik MT, Smith MS, Edelmann SE, et al. a 2 and a 1-Adrenergic receptors in the regulation of peripheral vascular function. In: “a 2-Adrenergic Receptors: Structure, Function and Therapeutic Implications”. Lanier SM and Limbird LE eds. Harwood Academic Publishers. 1997:171-178.
6. Trendelenburg AU, Limberger N, Rump LC. a 2-Adrenergic receptors of the a 2C subtype mediate inhibition of norepinephrine release in human kidney cortex. Mol Pharmacol 1994; 415:M68-M76.
7. Galitzky J, Larrouy D, Berlan M, Lafontan M. New tools for human fat cell alpha2A-adrenoceptor characterization. Identification on membranes and on intact cells using the agonist [3H]RX821002. J Pharmacol Exp Ther 1990; 252:312-319.
8. Lafontan M, Berlan M. Fat cell alpha2-adrenoceptors: the regulation of fat cell function and lipolysis. Endocr Rev 1995 Dec, 16(6):716-738.
9. Arner P, Kriegholm E, et al. Adrenergic regulation of lipolysis in situ at rest and during exercise. J Clinical Invest 1990; 85:893-898.
10. Maurige P, J Galitzky, M Berlan, M Lafontan. Heterogeneous distribution of beta and alpha-2 adrenoceptor binding sites in human fat cells from various fat deposits: functional consequences. Eur J Clin Invest 1987; 17:156-165.
11. Lafontan M, et al. Adrenergic regulation of adipocyte metabolism. Hum Reprod 1997 Oct; 12 Suppl 1:6-20.
12. Gether U, Lin S, Kobilka BK. Delineating ligand-specific structural changes in adrenergic receptors by use of fluorescence spectroscopy. In: “a 2-Adrenergic Receptors: Structure, Function and Therapeutic Implications”. Lanier SM and Limbird LE eds. 1997. Harwood Academic Publishers. 1997: 31-42.
13. Hodgetts V, Coppack S, Frayn KN, Hockaday TDR. Factors controlling fat mobilization from human subcutaneous adipose tissue during exercise. J Appl Phys 1991; 71:445-451.
14. Millet L, Barbe M, Lafontan M, Berlan M, Galitzky J. Catecholamine effects on lipolysis and blood flow in human abdominal and femoral adipose tissue. J Appl Physiol 1998; 85(1):181-188.
15. Ruffolo RR, Bondinell W, Hieble JP. a – and b -Adrenoceptors: From the gene to the clinic. 2. Structure-activity relationships and therapeutic applications. J Med Chem 1995; 38(19):3415-3444.
16. Arner P, Bolinder J. Microdialysis of adipose tissue. J Int Med 1991; 230:381-386.
17. Frayn KN, Fielding BA, Summers LKM. Investigation of human adipose tissue metabolism in vivo. J Endo 1997; 155:187-189.
18. Willette RN, Hieble JP, Sauermelch CF. The role of the alpha adrenoceptor subtypes in sympathetic control of the acralcutaneous microcirculation. J Pharmacol Exp Ther 1991; 256:599-605.
19. Borbujo J, Garcia-Villalon AL, Balle J, et al. Postjunctional alpha-1 and alpha-2 adrenoceptors in human skin arteries. An in vitro study. J Pharmacol Exp Ther 1989; 249:284-287.
20. Galitzky J, Lafontan M, Nordenstrom J, Arner P. Role of vascular alpha-2 adrenoceptors in regulating lipid mobilization from human adipose tissue. J Clin Invest 1993; 91:1997-2003.
21. Horn PT, Kohli JD, Listinsky JJ, Goldberg LI. Regional variation in the alpha-adrenergic receptors in the canine resistance vessels. Nauyn-Schmiedeberg’s Arch Pharmacol 1982; 318:166-172.
22. Flavahan NA, Cooke JP, Shepherd JT, Vanhoutte PM. Human postjunctional alpha-1 and alpha-2 adrenoceptors: differential distribution in arteries of the limbs. J Pharmacol Exp Ther 1987; 24:361-365.
23. Glusa E, Markwardt F. Characterization of postjunctional alpha-adrenoceptors in isolated human femoral veins and arteries. Nauyn-Schmiedeberg’s Arch Pharmacol 1983; 323:101-105.
24. Enoksson S, Nordenstrom J, Bolinder J, Arner P. Influence of local blood flow on glycerol levels in human adipose tissue. Int J Obesity 1995; 19:350-354.
25. Kovach AGB, Kovach E, Sandor P, et al. Metabolic responses to localized ischemia in adipose tissue. J Surg Res 1976; 20:37-44.
26. Barbe P, Galitzky J, Riviere D, Senard JM, et al. Effects of physiological and pharmaceutical variation of sympathetic nervous system on plasma non-esterified fatty acid concentrations in man. Br J Pharm 1993; 36:25-30.
27. Harmelen VV, Lonnqvist F, Thorne A, et al. Noradrenaline-induced lipolysis in isolated mesenteric, omental and subcutaneous adipocytes from obese subjects. Int J Obesity 1997; 21:972-979.
28. Arner P. Regulation of lipolysis in fat cells. Diab Rev 1996; 4:450-463.
29. Bjorntorp P. Obesity and the adipocyte. Neuroendocrine factors in obesity. J Endocrin 1997; 155:193-195.
30. Abate N, Garg A. Heterogeneity in adipose tissue metabolism: causes, implications, and management of regional adiposity. Prog Lipid Res 1995; 34:53-70.
31. Maurige P, et al. Regional difference in adipose tissue lipolysis from lean and obese women: existence of postreceptor alterations. Am J Physiol 1995 Aug; 269(2 pt 1): E341-E350.
32. Hellstrom L, Blaak E, Hagstrom-Toft E. Gender differences in adrenergic regulation of lipid mobilization during exercise. Int J Sports Med 1996; 17:439-447.
33. Goldberg MR Robertson D. Yohimbine: a pharmacological probe for study of the a 2-adrenoceptor. Pharmacol Rev 1983;35:143-180.
34. Berlan M, Galitzky J, Riviere D, et al. Plasma catecholamine levels and lipid mobilization induced by yohimbine in obese and non-obese women. Int J Obesity 1991; 15:305-315.
35. Galitzky J, Taouis M, Berlan M, Riviere D, et al. a 2-Antagonist compounds and lipid mobilization: evidence for a lipid mobilizing effect oral yohimbine in healthy male volunteers. Eur J Clin Invest 1988; 18:587-594.
36. Galitzky j, Riviere D, Tran MA, Montastruc JL, Berlan M. Pharmacodynamic effects of chronic yohimbine treatment in healthy volunteers. Eur J Clin Pharmacol 1990; 39:447-451.
37. Kuchio C, Jonderdo K, Piskorska D. Does yohimbine act as a slimming drug? Isr J Med Sci 1991; 27:550-556.
38. Zahorska-Markiewiz B, Kuchio, Piskorska D. Adrenergic control of lipolysis and metabolic responses in obesity. Horm Metabol Res. 1986; 18:693-697.
39. Murburg MM, Villacres EC, Ko BN, Veith RC. Effects of yohimbine on human sympathetic nervous system function. J Clin Endocrin Metab 1991; 73:861-865.
40. Sax L. Yohimbine does not affect fat distribution in men. Int J Obesity 1991; 3:261-280.
41. Bulow J. Adipose tissue blood flow during exercise. Dan Med Bull 1983; 30:85-100.
42. Bulow J, Madsen J. Regulation of fatty acid mobilization from adipose tissue during exercise. Scand J Sports Sci 1986; 8:19-26.
43. Bulow J, Madsen J, Astrup A, Christensen NJ. Vasoconstrictor effect of high FFA/albumin ratios in adipose tissue in vivo. Acta Physiol Scand 1985; 125:661-667.
44. Berlan M, Montastruc JL, Lafontan M. Pharmacological prospects for a 2-adrenoceptor antagonist therapy. TiPS Rev 1992; 13:277-282.
45. Montastruc P, Belan M, Monstastruc JL. Effect of yohimbine on submaxiallary salivation in dogs. Br. J Pharmac 1989; 98:101-104.
46. Chatelut E, Rispail Y, Berlan M, Montastruc JL. Yohimbine increases human salivary secretion. Br J Clin Pharmac 1989; 366-368.)
47. Fiaramonti J, Berlan M, Fargeas MJ, Bueno L. Yohimbine stimulates colonic motility through a central action in conscious dogs. J Gastrointes Mot 1992; 4:137-141.
48. Charney DS, Heninger GR, Breier A. Noradrenergic function in panic anxiety. Effects of yohimbine in healthy subjects and patients with agoraphobia and panic disorder. Arch Gen Psychiatry 1984; 41:751-763.
49. Murburg MM, Villacres EC, Ko BN, Veith RC. Effects of yohimbine on human sympathetic nervous system function. J Clin Endocrin Metab 1991; 73:861-865.
50. Grunhaus L, Tiongco D, Zelnik T, Flegel P, et al. Intravenous yohimbine. Selective enhancer of norepinephrine and cortisol secretion and systolic blood pressure in humans. Clin Neuropharmacol 1989; 12:106-114.
51. Henninger GR, Charney DS, Price LH. a 2-Adrenergic receptor sensitivity in depression. The plasma MHPG, behavioral and cardiovascular responses to yohimbine. Arc Gen Psychiatry 1988; 45:718-726.
52. McDougle CJ, Krystal JH, Price LH, Heninger GR, et al. Noradrenergic response to acute ethanol administration in healthy subjects: comparisons with intravenous yohimbine. Psychopharmacol 1995; 118:127-135.
53. Betz, JM, White KD. Gas chromatographic determination of yohimbine in commercial yohimbine products. J AOAC Int. 1995; 78:1189-1194.
54. Benedek IH, Blouin RA, McNamara PJ. Serum protein binding and the role of increased alpha1-acid glycoprotein in moderately obese subjects. Br J Clin Pharmacol 1984; 18:941-946.
55. Guthrie SK, Hariharan M, Grunhaus LJ. Yohimbine bioavailability in humans. Eur J Clin Pharmacol 1990; 39:409-411.
56. Hedner T, Edgar B, Edvinsson L, Hedner J, et al. Yohimbine pharmacokinetics and interaction with the sympathetic nervous system in normal volunteers. Eur J Clin Pharmacol 1992; 43:651-656.
57. Owen JA, Hakatsu SL, Fenemore J, Condra M, et al. The pharmacokinetics of yohimbine in man. Eur J Clin Pharmacol 1987; 32:577-582.
58. Le Verge R, Le Corre P, Chevanne F. Determination of yohimbine and its two hydroxylated metabolites in humans by high-performance liquid chromatography and mass spectral analysis. J Chromatog 1992; 574:283-292.
59. Brannan T, Martinez-Tica J, Yahr MD. Effect of yohimbine on brain monoamines: an in vivo study. J Neural Transm 1991; 3:81-87.
60. Hubbard JW, Pfister SL, Beidinger Am, Herzig TC, et al. The pharmacokinetic properties of yohimbine in the conscious rat. Naunyn-Schmiedeberg’s Arch Pharmacol 1988; 337:583-587.
About the author
Elzi has spent the last several decades trying to determine where 'home' is: from New York, Maine, California, Oregon and now Texas. As well, her career has encompassed tool & die apprentice, forest ranger, assistant extension agent, mother, sheep and horse rancher, and mad research scientist. She has also been a competitive bodybuilder, but has found true joy in powerlifting. When Elzi is not playing fetch with her 1200 lb four-footed buddy, she is most happy in the gym and in a research lab.
Leave a Reply
You must be logged in to post a comment.