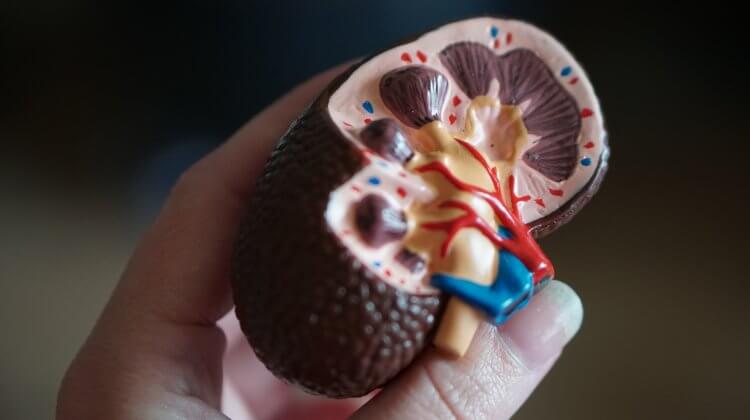
The kidneys are responsible, among other things, for filtering the blood and producing urine. They do so by creating a filtrate from the large amounts of blood that are flowing through them. Usually, over a liter of blood passes through the kidneys every minute. If you subtract the nonfluid fraction of this, thus leaving you with the blood plasma, this translates to roughly 625 mL of blood plasma that flows through the kidneys every minute. About one fifth of this fluid gets squeezed through the glomerular capillaries (see picture below) in every single nephron of which the kidneys consist of. A nephron is the functional unit of the kidney. Each is capable of filtering the blood and producing what’s ultimately urine. A kidney consists of roughly 1 million nephrons, but there’s considerable variation in this from one person to another [1]. The fluid that’s squeezed through the glomerular capillaries is captured in a ‘sack’ called Bowman’s capsule. The rate at which this fluid, or glomerular filtrate, is collectively captured in Bowman’s capsule by all the nephrons per minute is what is referred to as the glomerular filtration rate (GFR). In healthy adults, this is around 125 mL/min (20% of the above mentioned 625 mL/min).
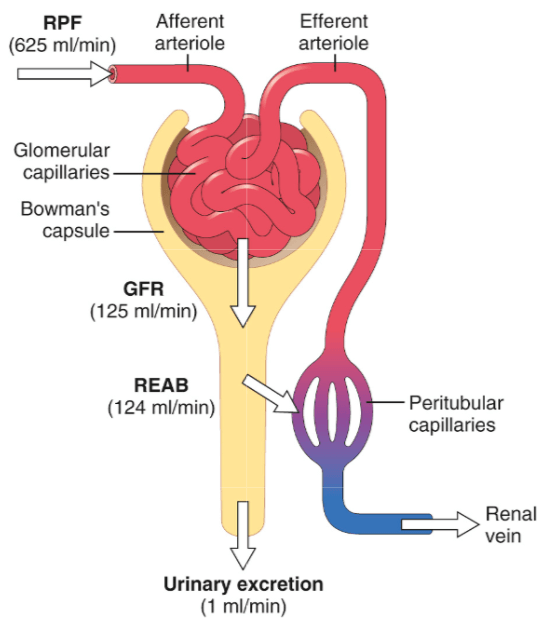
Estimating the glomerular filtration rate (eGFR)
Estimation of the GFR is used as an indicator of kidney function. The best method of doing so is by using a substance that is freely filtered at the glomerulus and neither secreted, reabsorbed, synthesized, nor metabolized by the kidney. As such, whatever amount of the substance gets filtered at the glomerulus also gets excreted in the urine. And, as such, the GFR can be accurately derived from the urine measurements and knowing how much you’re administering. The gold standard to measure it is by using a substance called inulin. It’s hardly used in practice because it’s expensive, most labs can’t assay it and for the most accurate assessment it’d require an IV with multiple blood samples and catheterization of the bladder. All in all, not very practical.
As such, the GFR is often estimated based on the serum creatinine concentration. Creatinine doesn’t get reabsorbed or metabolized by the kidneys and is freely filtered at the glomerulus. Additionally, there’s a constant rate of supply from the skeletal muscle tissue (as it’s a breakdown product of creatine) so nothing needs to be IV’ed—contrary to inulin. However, there can be significant tubular secretion of creatinine [2]. Thus, while not being absolutely perfect, these properties of creatinine still make it useful to derive the GFR from. Several formulas have been established that can provide an estimate of the GFR (eGFR) based on its concentration. All are based on the assumption that higher creatinine levels imply that less creatinine is being cleared, i.e. a decreased GFR.
The current formula that is being recommended in clinical practice is the Chronic Kidney Disease Epidemiology Collaboration (CKD-EPI) equation [3]. Before this, the Modification of Diet in Renal Disease (MDRD) was commonly used. The CKD-EPI equation takes, in addition to the serum creatinine concentration, sex, age, and race into account and is corrected to a body surface area of 1.73 m2. This will give eGFR values with a unit of mL/min/1.73m2. A normal or high eGFR is considered to be greater than 90 [3].
Issues with the eGFR based on creatinine in anabolic steroid users
Estimating the GFR based on the serum creatinine concentration is notoriously unreliable in muscular individuals. Since muscle is the primary storage site of creatine in the body, muscular individuals will carry around more creatine in their bodies. Consequently, the rate of creatinine production is also higher. As such, with similar clearance rates, the serum creatinine levels will also be higher. As a result, these formulas will underestimate the true GFR. Another issue that arises in this population is one caused by creatine supplementation. Creatine supplementation is common practice among resistance-trained individuals and is effective in increasing body stores of creatine [4]. As such, there’s also an absolute higher rate of creatinine production. Moreover, creatine ethyl ester in particular can lead to sharp increases in serum creatinine levels [5, 6]. The most likely reason for this is that creatine ethyl ester is rapidly degraded into creatinine in the body after ingestion [7]. (Also rendering it rather useless as a supplement.) The ingestion of cooked meat can also transiently increase serum creatinine levels for several hours [8]. Additionally, there’s evidence indicating that anabolic steroid use might increase the biosynthesis of creatine. Creatine is synthesized by a two-step mechanism as pictured below:

The reaction catalyzed by AGAT, forming guanidinoacetic acid, is the rate-limiting step in the synthesis of creatine [9]. It has been found that administration of an anabolic steroid (17α-methyl testosterone) increases AGAT expression [10]. Moreover, it increased the excretion of guanidinoacetic acid in the urine by 70 %. Taken together this is strongly suggestive of, at least oral bioavailable, anabolic steroids stimulating the biosynthesis of creatine. Resultantly, they might increase creatine storage and thereby also affect the absolute rate of creatinine production. Finally, most anabolic steroid users are also consuming a high-protein diet. A high-protein diet has been found to increase the GFR [11]. Note that this is not an overestimation of the GFR, it actually increases the true GFR a bit.
Summarized, the following things can affect the serum creatinine levels and thereby the estimated GFR without actually affecting the true GFR:
- Being more muscular
- Supplementing creatine (especially creatine ethyl ester)
- Having eaten cooked meat in the hours prior to measurement
- Taking (oral) anabolic steroids
Having said that, if you keep into account that the eGFR will be underestimated by these factors, you can, of course, still observe changes in the eGFR over time. Assuming you keep everything quite constant, these changes can be indicative of changes in the glomerular filtration rate.
Anabolic steroids affect creatinine-based eGFR
Few trials have measured the effect of anabolic steroids, testosterone enanthate in particular, on serum creatinine levels. Bhasin et al. reported a minor increase from 1.0 mg/dL to 1.1 mg/dL in normal men receiving 600 mg testosterone enanthate weekly in conjunction with resistance exercise [12]. However, a later study by the same group didn’t detect any significant changes in serum creatinine levels in healthy young men receiving graded doses of testosterone enanthate from 25 up to 600 mg weekly for a duration of 20 weeks [13]. The same group, again with a similar study design, but in older men, did find an increase from 1.03 to 1.17 mg/dL in the men receiving 600 mg weekly and from 1.12 to 1.19 mg/dL in the group receiving 125 mg weekly (which is close to a testosterone replacement therapy [TRT] dosage) [14]. A trial which provided the oral prohormone 1-androsterone in a dosage of 330 mg daily for 4 weeks also noted an increase from 1.1 mg/dL to 1.3 mg/dL in serum creatinine levels [15]. The authors also calculated the eGFR, which dropped from 88.3 to 71.9 m?l/min/1.73m2. It’s unclear whether these increases in serum creatinine reflect an actual decrease in GFR or are simply artifacts arising from the issues pertaining creatinine-based eGFR as outlined earlier. Notably, kidney dysfunction or disease is not particularly known to be caused by anabolic steroids with the exception of some case reports.
Alternatives to creatinine-based eGFR
In cases in which there is clear reason to suspect that the eGFR based on serum creatinine levels is inaccurate, some alternative methods might be used. One of them is based on measurement of serum cystatin C levels. The idea is roughly similar to that of creatinine measurements. The main difference is that cyostatin C is produced by all (nucleated) cells at a relatively constant rate. However, an important difference is that some metabolization of the substance does occur in the tubules. Moreover, while originally assumed to not be affected by gender, age or muscle mass, accumulating evidence suggests it actually does. Several studies have found it to be affected by gender, age, race, weight, height, body composition and smoking status [16, 17, 18]. Nevertheless, one study concluded that cystatin C might represent a more adequate alternative to assess renal function in individuals with higher muscle mass when mild kidney impairment is suspected [19]. It’s likely that eGFR based on cystatin C might yield a clearer picture of the true GFR than eGFR based on creatinine does in resistance-trained individuals who do or do not use anabolic steroids. Finally, data suggests that combining the two measurements might even paint an even more accurate picture than either alone in chronic kidney disease [20]. However, this data has not been verified in muscular individuals/bodybuilders specifically.
In research studies more reliable markers such as iothalamate and iohexol are also used. While both can be used with a single bolus injection, they do require multiple plasma measurements. Nevertheless, they’re both inexpensive and yield better estimates than creatinine/cystatin C-based eGFR. Iothalamate is—as far as I know—used the least in clinical practice and is radioactive (iohexol isn’t). Iohexol does carry a small risk of nephrotoxicity and risk of allergic reaction (especially at high doses). I’m mentioning this more for completeness rather than anything else, as it’s not something that should routinely be used.
Focal segmental glomerulosclerosis (FSGS) and detecting kidney damage with urine measurements
There are very little reports in the literature about anabolic steroids being damaging to the kidneys. A notable paper that reports about kidney dysfunction in anabolic steroid users is a 2010 paper by Herlitz et al. [21]. It describes 10 patients from the archives of their renal pathology laboratory over a 10-year-period. The patients were all bodybuilders with a long history of anabolic steroid use. Renal biopsies were taken which revealed focal segmental glomerulosclerosis (FSGS) in nine of them, and four of them also had glomerulomegaly. In one of the patients they didn’t find signs of FSGS but only detected glomerulomegaly. So what is FSGS? It’s a bit of an umbrella term for a group of diseases which leads to glomerular lesions, mediated by diverse insults directed to, or inherent within, the podocyte (the cells forming the outer surface of the glomerular capillaries) [22]. Or perhaps better said, it’s a histological finding without necessarily indicating a specific disease. Either way, in FSGS, the podocytes start to change their shape, becoming sort of flattened (effacement). At some point, the podocyte ultimately dies and detaches from the basement membrane. Since podocytes are what is called ‘terminally differentiated’, they can’t undergo cell division (proliferate). As such, these cells get replaced with connective tissue (sclerosis). Obviously, connective tissue doesn’t function like the podocytes do and as such the filtering function of the glomerulus is compromised.
This can manifest itself in the loss of protein in the urine. Which shouldn’t be there (except for some trace amounts). The bodybuilders in this study lost large amounts of protein in their urine (on average around 10 grams daily). For perspective, this should usually be not more than a few milligrams. One of the bodybuilders was even capable of producing his own protein shake, as he peed out a freaking 26 g of protein daily. Notably, serum creatinine was also markedly elevated in these subjects. While the normal range spans from 0.9 mg/dL to 1.3 mg/dL, these subjects showed on average serum creatinine levels of 3.0 mg/dL, with one as high as a whopping 7.8 mg/dL. Obviously, these large deviations are clearly caused by a decrease in kidney function.
There are a few things I would like to mention about this paper. One being that these bodybuilders weren’t your average bodybuilders. Their BMI on average was 35 kg/m2. They were pretty damn huge. Four of them admitted to using anabolic steroids in conjunction with GH, and one of them with insulin. Additionally, six also had hypertension. Below is a picture of one of them:
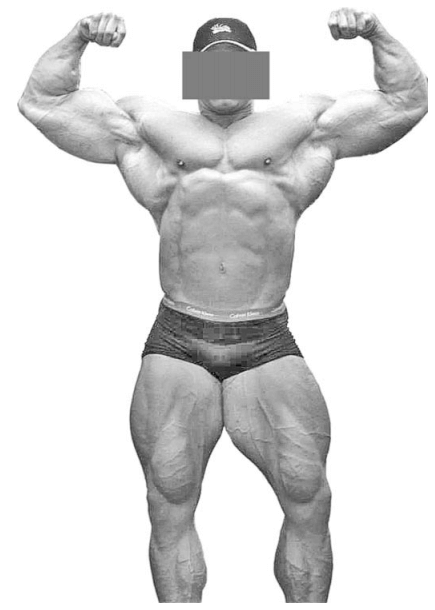
They managed to do a follow-up of eight of the subjects after they had stopped anabolic steroids (and all but one were also prescribed medication, mostly stuff that inhibits the renin-angiotensin-aldosterone system [RAAS]). At the follow-up, great improvements were seen in proteinuria, and variable improvements were seen in serum creatinine levels. Notably, one patient relapsed into using anabolic steroids again and saw his protein excretion in urine vastly increase again.
Taken together, while it’s hard to say that all of this could be the result of anabolic steroid usage, it does seem probable that in some rare cases excessive chronic anabolic steroid use might lead up to this. Also because you don’t see much of this in the literature, despite the millions and millions of anabolic steroid users about the globe. However, there might be some significant underreporting, as milder forms of kidney damage might go unnoticed, even with the routine measurements of eGFR. Early detection might be achieved with testing for protein in the urine, which simply rarely is done without indication.
As such, it might be recommended to do urine measurements somewhat regularly. For example, annually or biannually. Slight elevations of albumin in the urine should prompt for a retest, as this can also result from for example infection or exercise, without being caused by actual kidney damage. With persistent elevations or large elevations further follow up should be initiated and, ideally, anabolic steroid usage should be stopped.
References
- Bertram, John F., et al. “Human nephron number: implications for health and disease.” Pediatric nephrology 26.9 (2011): 1529-1533.
- Shemesh, Ovadia, et al. “Limitations of creatinine as a filtration marker in glomerulopathic patients.” Kidney international 28.5 (1985): 830-838.
- Eknoyan, Garabed, et al. “KDIGO 2012 clinical practice guideline for the evaluation and management of chronic kidney disease.” Kidney Int 3.1 (2013): 5-14.
- Harris, Roger C., Karin Söderlund, and Eric Hultman. “Elevation of creatine in resting and exercised muscle of normal subjects by creatine supplementation.” Clinical science 83.3 (1992): 367-374.
- Williamson, Lydia, and David New. “How the use of creatine supplements can elevate serum creatinine in the absence of underlying kidney pathology.” Case Reports 2014 (2014): bcr2014204754.
- Velema, M. S., and W. De Ronde. “Elevated plasma creatinine due to creatine ethyl ester use.” Neth J Med 69.2 (2011): 79-81.
- Gufford, Brandon T., et al. “pH-dependent stability of creatine ethyl ester: relevance to oral absorption.” Journal of dietary supplements 10.3 (2013): 241-251.
- Preiss, David J., et al. “The influence of a cooked-meat meal on estimated glomerular filtration rate.” Annals of clinical biochemistry 44.1 (2007): 35-42.
- Walker, James B. “Creatine: biosynthesis, regulation, and function.” Adv Enzymol Relat Areas Mol Biol 50.177 (1979): 2.
- Hoberman, Henry D., Ethan AH Sims, and William W. Engstrom. “The effect of methyltestosterone on the rate of synthesis of creatine.” Journal of Biological Chemistry 173.1 (1948): 111-116.
- Martin, William F., Lawrence E. Armstrong, and Nancy R. Rodriguez. “Dietary protein intake and renal function.” Nutrition & metabolism 2.1 (2005): 1-9.
- Bhasin, Shalender, et al. “The effects of supraphysiologic doses of testosterone on muscle size and strength in normal men.” New England Journal of Medicine 335.1 (1996): 1-7.
- Bhasin, Shalender, et al. “Testosterone dose-response relationships in healthy young men.” American Journal of Physiology-Endocrinology And Metabolism (2001).
- Bhasin, Shalender, et al. “Older men are as responsive as young men to the anabolic effects of graded doses of testosterone on the skeletal muscle.” The Journal of Clinical Endocrinology & Metabolism 90.2 (2005): 678-688.
- Granados, Jorge, et al. “Prohormone supplement 3β-hydroxy-5α-androst-1-en-17-one enhances resistance training gains but impairs user health.” Journal of applied physiology 116.5 (2014): 560-569.
- Knight, Eric L., et al. “Factors influencing serum cystatin C levels other than renal function and the impact on renal function measurement.” Kidney international 65.4 (2004): 1416-1421.
- Groesbeck, Darcy, et al. “Age, gender, and race effects on cystatin C levels in US adolescents.” Clinical Journal of the American Society of Nephrology 3.6 (2008): 1777-1785.
- Macdonald, Jamie, et al. “GFR estimation using cystatin C is not independent of body composition.” American journal of kidney diseases 48.5 (2006): 712-719.
- Baxmann, Alessandra Calábria, et al. “Influence of muscle mass and physical activity on serum and urinary creatinine and serum cystatin C.” Clinical Journal of the American Society of Nephrology 3.2 (2008): 348-354.
- Stevens, Lesley A., et al. “Estimating GFR using serum cystatin C alone and in combination with serum creatinine: a pooled analysis of 3,418 individuals with CKD.” American journal of kidney diseases 51.3 (2008): 395-406.
- Herlitz, Leal C., et al. “Development of focal segmental glomerulosclerosis after anabolic steroid abuse.” Journal of the American Society of Nephrology 21.1 (2010): 163-172.
- D’Agati, Vivette D., Frederick J. Kaskel, and Ronald J. Falk. “Focal segmental glomerulosclerosis.” New England Journal of Medicine 365.25 (2011): 2398-2411.
Main photo by Robina Weermeijer on Unsplash
About the author
Peter Bond is a scientific author with publications on anabolic steroids, the regulation of an important molecular pathway of muscle growth (mTORC1), and the dietary supplement phosphatidic acid. He is the author of several books in Dutch and English, including Book on Steroids and Bond's Dietary Supplements.
12 replies
Loading new replies...
Join the full discussion at the MESO-Rx →