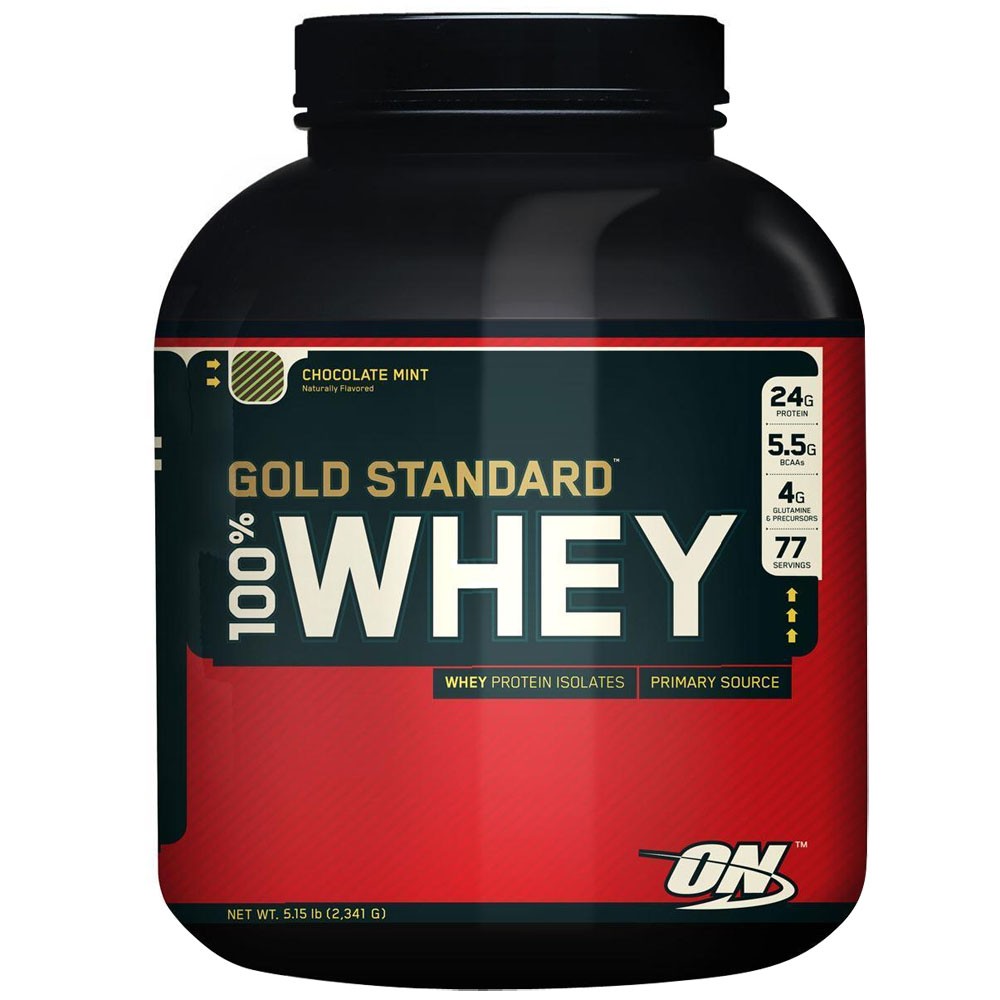
This article is the second in a series of articles discussion the details of protein metabolism in bodybuilders. Part 1 addressed some basic concepts and definitions regarding protein, digestion of different forms of protein as well as total protein requirements for athletes. Part 2 of this article series builds on Part 1 with a discussion of protein digestibility and the beginning of a discussion of protein quality, which will be continued in detail in Part 3.
Section 4: Protein digestibility
An important aspect of protein metabolism is how well or how poorly a given protein is digested by the human body. Claims are sometimes made that protein powders (especially predigested or hydrolyzed proteins) are digested more efficiently than whole food proteins. Claims are also occasionally made that vegetable source proteins are more digestible than animal-based. Protein digestibility is measured by seeing how much nitrogen is excreted in the feces compared to the amount of nitrogen which is ingested. A correction is made for the amount of nitrogen which is normally lost in the feces. Therefore, digestibility research examines how much more nitrogen is lost over normal levels when a given protein is fed. If an individual were fed 5 grams of nitrogen (approximately 30 grams of protein) and 1 gram of nitrogen was excreted in the feces, this would represent a digestibility of 80% (4 grams retained divided by 5 grams consumed). Table 1 shows the digestibility for some common proteins.
Table 1: Digestibility of common proteins foods
Food source | Protein digestibility (%) |
Egg | 97 |
Milk and cheese | 97 |
Mixed US diet | 96 |
Peanut butter | 95 |
Meat and fish | 94 |
Whole wheat | 86 |
Oatmeal | 86 |
Soybeans | 78 |
Rice | 76 |
Source: National Research Council. Recommended Dietary Allowances, 10th ed. National Academy Press, 1989.With the exception of some plant-based proteins, we see that most commonly eaten proteins (e.g. those eaten by bodybuilders) have extremely high digestibilities. Assuming that someone has a normally functioning digestive tract, there is no reason to think that a protein powder will digest significantly better or have a greater impact on growth than a whole food protein (2). Even if a given protein powder did achieve a true digestion of 100% (unlikely, since no process in the body is 100% efficient), this would only mean a 3% difference compared to egg or milk protein. In practical terms, for every 100 grams of protein eaten, there would be a 3 gram difference in intake. While this might be of importance at low levels of protein intake, at the kinds of protein intakes seen in bodybuilders, slight differences in digestibility are unlikely to have a great impact on growth. Therefore the primary difference between a protein powder and a whole food protein will not be in how well one is digested relative to the other, but in how quickly they will digest. As discussed in Part 1 of this article, the predigested nature of many protein powders will speed digestion and release into the bloodstream.
Section 5: Protein quality
Protein quality is a topic of major debate, both in the research world, as well as in the realm of protein supplements. Arguments have been made that one protein is of higher quality than another, or that protein powders are superior to whole food protein. Since this is an area of such debate, the issue of protein quality will be discussed in some detail. Protein quality refers, in a general sense, to how well or poorly a given protein will be used by the body. More specifically, it refers to how well the indispensable amino acid (AA) profile of a protein matches the requirements of the body (3). However, this should not suggest that the content of dispensable AAs in a protein is irrelevant to protein quality as the body. As we will see below, the problem of AA requirements is not an easy one to solve, making arguments about protein quality even more problematic. Methods of measuring protein quality There are a variety of methods available to measure protein quality. To a great degree, how a protein’s quality is rated depends on what method is used. This is part of what allows protein sellers to argue the superiority of one protein over another. For example, measured by one method, egg protein may be the highest quality protein, but by another method, casein may be the highest. Additionally, and perhaps more importantly, the quality of a protein is directly related to the physiological needs of the subject being studied (3). The protein that is optimal for a bodybuilder in a mass phase may not be the same as the protein that is optimal while dieting or for an endurance athlete. Diet and activity can affect how AAs are used in the body. For example, long-duration endurance activity tends to oxidize high quantities of the branch-chain amino acids (BCAAs) (4), suggesting that endurance athletes might have a higher BCAA requirement than non-endurance athletes. In all likelihood, there is no single protein that can be rated as the highest quality for all situations. So the first question which has to be answered is which method of rating proteins is ideal for humans. The short answer is that none of them are ideal, since all make assumptions, or are based on models that may or may not be accurate. The second question, which no one has addressed, is whether the AA requirements of a sedentary individual is the same or different than that of a hard training bodybuilder. Although there are numerous different methods to compare proteins, only a few are used frequently enough in popular literature to require discussion. They are: chemical score, biological value, protein efficiency ratio, and protein digestibility corrected amino acid score. Chemical score (5) Chemical score is method of rating proteins based on it’s chemical composition (more specifically it’s indispensable AA levels). To determine chemical score, a protein is picked as a reference and other proteins are rated relative to that reference protein. This is conceptually similar to giving white bread a value of 100 on the glycemic index scale and rating other carbohydrates relative to that. Typically, egg protein has been used as the reference protein, but this assumes that the amino acid profile of egg is the ideal for humans. Recently, other amino acid patterns have been suggested to replace egg based on increasing information about the AA requirements of humans. In 1985, a joint committee on protein requirements suggested an idealized reference pattern (6), but this pattern has been criticized by many researchers as being too low (for example 7,8). Since chemical score is a relative, and not an absolute scale, it is possible to have values greater than 100. If 5 grams of the reference protein contains 800 mg of a certain amino acid, and 5 grams of the test protein contains 1000 mg of that same amino acid, the second protein would be rated as 125% for that amino acid. The indispensable amino acid present in the lowest quantity (relative to what is required) is defined as the first limiting amino acid (see Part 1 for a discussion of limiting amino acids). The second lowest indispensable AA relative to requirements would be the second limiting amino acid, etc. In general, the limiting amino acid will determine how well or how poorly a given protein is used by the body. This suggests that supplementation of the limiting amino acid (i.e. taking additional methionine with a protein which is limited by methionine) or combining proteins with different limiting amino acids might increase the quality of the protein. Chemical score can also be used to compare a given protein to the amounts required by the subject in question. This is somewhat more useful in that it takes into account the needs of the individual, assuming they are known. That is, if a given protein provided 100 mg/kg of a certain AA, and 150 mg/kg of that AA were required by an individual, the chemical score would be 0.67 for that amino acid (meaning that the protein in question provided only 67% of the amount required by that person). While chemical score is useful for rating proteins based on their composition, it has one major drawback: it has little to do with how a food protein will be used in the body (human or otherwise) since it does not take into account digestibility. For this reason, chemical score is rarely the only measure of protein quality used to rate a protein. Biological value (BV) (5) Biological value (BV) is probably one of the most commonly used measures of a protein’s quality. The BV of a protein is given as the amount of nitrogen retained in the body divided by the amount of nitrogen absorbed from that protein. Therefore, digestibility of that protein is taken into account. Thus: BV = (nitrogen retained / nitrogen absorbed) * 100 A BV of 100 would indicate complete utilization of a given dietary protein, in that 100% of the protein ingested was stored in the body with none lost. To measure BV, subjects are typically fed a zero protein diet so that baseline losses of nitrogen can be measured (i.e. the amount of nitrogen that is lost normally). Then the test protein is fed at varying levels (generally 0.6, 0.5, 0.4 and 0.3 g/kg are fed) and a nitrogen balance study is done (9). Some studies use longer periods of starvation and this is an important consideration in evaluating the data. For example, the study often cited by advertisers to demonstrate the ‘superiority’ of whey protein hydrosylate measured nitrogen balance in rats after three days of starvation, which corresponds to a longer period in humans (10). In this study, whey protein hydrosylate led to better nitrogen retention and growth than the other proteins studied. What is not mentioned is that starvation affects how well the body will store incoming protein, leading to falsely elevated BV measures. This study has little bearing to an individual with a habitual high-protein intake. A full discussion of the effects of low protein eating (i.e. protein cycling) will appear in Part 3 of this article series. Although nitrogen balance methodology has it’s problems (see Part 1 of this article series), it is a rough indicator of how well or poorly a given protein supports the body’s needs. If a given amount of protein (more accurately, a given amount of nitrogen) places an individual in nitrogen balance (or positive nitrogen balance) it can be assumed that the protein in question is of sufficient quality to support maintenance of body protein stores. The biggest drawback of the nitrogen balance method is that it gives no information regarding specific amino acid metabolism (and deficiencies) or the specific tissues which are being affected (e.g. muscle vs. liver), only an indication of what is occurring on the whole body level (9). Depending on the individual amino acid requirements of a given tissue, it is possible that a protein might optimally support protein synthesis in one organ, such as the liver, while not optimally supporting synthesis in another tissue, such as muscle. The issue of whole body versus specific tissue protein metabolism will be discussed in Part 3 of this series. Despite what is sometimes claimed, it is impossible to have a BV greater than 100. Additionally, there is no indication that the percentage sign was ever dropped from the BV measure. For example, it’s been suggested that whey protein has a BV of 157, but this would imply that 1.57 grams of nitrogen were stored for every 1 gram of nitrogen consumed. Since it is thermodynamically impossible for the body to store more nitrogen than was ingested, a BV of 157 is equally impossible. Protein advertisements claiming BV higher than 100 should be looked upon with suspect. One aspect of measuring BV that can cause problems in interpretation of results is that the BV of a protein is affected by a number of factors. The first of these is caloric intake. A very high caloric intake will improve nitrogen balance at any given protein intake and vice versa. This means that an individual consuming a lot of calories (e.g. a bodybuilder on a mass-gaining diet) will show improved nitrogen retention and ‘apparent’ BV will go up (i.e. more nitrogen will be retained compared to the amount eaten). By the same token, if calories are decreased (e.g. during a diet), BV will go down. A secondary factor which affects BV is activity. Exercise, especially weight training, increases nitrogen retention which will give a protein a higher apparent BV. A third factor, and one that is typically ignored in popular literature is that the BV of a protein is related to the amount of protein given (9). BV is measured at levels below the maintenance level. As protein intake goes up, the BV of that protein goes down. For example, milk protein shows a BV near 100 at intakes of 0.2 g/kg. As protein intake increases to roughly maintenance levels, 0.5 g/kg, BV drops to 70 or so (9). To quote from Pellett and Young, “….protein is utilized more effectively at suboptimal levels than at levels in the near-maintenance range of intake. Accordingly biological measures of protein quality conducted at suboptimal levels in either experimental animals or human subjects may overestimate protein value at maintenance levels.” (9) Therefore, while BV may be important for rating proteins where intake is below requirements, BV has little bearing on individuals with protein intakes far above requirements. Table 2 presents the BV of some common proteins. Table 2: BV of some common proteins
Protein | BV |
whey | 100? |
egg | 100 |
milk | 93 |
rice | 86 |
casein, fish and beef | 75 |
corn | 72 |
peanut flour | 56 |
wheat gluten | 44 |
Source: Normal and Therapeutic Nutrition, 17th ed. Corinne H. Robinson, Marilyn R. Lawler, Wanda L. Chenoweth, and Anne E. Garwick. Macmillan Publishing Company, 1986. Considering the high protein intakes of most strength athletes (2.0 g/kg or higher) it is hard to see how BV will play a meaningful role in rating proteins in this population. In all likelihood, any decent quality protein will be as good as any other at these types of protein intakes. Additionally, even if proteins such as whey have slightly higher BV ratings than protein sources like casein (milk) or egg, such a small difference is unlikely to affect mass gains in the long run. Protein efficiency ratio (PER) (5) PER is sometimes used to rate proteins and represents the amount of weight gained (in grams) relative the amount of protein consumed (in grams). For example, a PER of 2.5 would mean that 2.5 grams of weight was gained for every gram of protein ingested. Since it is impossible to measure weight gain in grams in humans, PER is generally measured in young, growing animals placed on a diet which is 10% protein by weight. This begs the question of whether young animals, who are growing, provide a good model for adult humans. While the Food and Drug Administration has suggested the use of PER with casein as a reference model for labeling protein foods (12), the use of PER to estimate human protein requirements has been criticized by some authors (13). While the use of PER to rate proteins for humans is debatable, it should be noted that a recent animal study found that combinations of animal (30% of total) and plant based proteins (70% of total) had a higher PER value than the animal or vegetable proteins eaten alone (14). This may have to do with the proteins ‘combining’ to decrease the impact of the limiting AA. Individuals who wish to decrease their intake of animal-based proteins may be able to achieve higher PER values with a combination of animal and plant based proteins than someone eating only animal based proteins. Protein digestibility corrected amino acid score (PDCAAS)PDCAAS is the newest method of protein quality to be developed. It has also been suggested as the ideal scale to rate proteins for their ability to meet human requirements (15). Similar to chemical score, it rates protein foods relative to a given reference protein. In this case, the AA profile used is that one determined to be ideal for children two to five years old as its reference protein for adults (15). This obviously raises the immediate question of how much relevance this AA profile has to adult bodybuilders. PDCAAS goes beyond chemical score, however, by factoring in the digestibility of a given protein, giving the AA profile more relevance to human needs. Interestingly, using the PDCAAS method, along with the proposed AA reference patter, proteins which were previously rated at poor quality, such as soy, have obtained higher quality ratings (16). This is more in line with studies showing that certain purified soy proteins, such as Supro ™ which is found in Twinlab Vege-fuel, can maintain adults in nitrogen balance (3,16). Once again, whether the use of PDCAAS to rate proteins for adult bodybuilders is debatable since the physiology of weight training may affect requirements for certain amino acids (i.e. glutamine, BCAAs).
Summary of protein quality
Although a variety of methods of measuring protein quality have been proposed, none are perfect in rating proteins for human use. While some methods of rating protein are based on how well (or poorly) an animal grows (or the nitrogen balance which is attained), these methods provide no information on specific amino acid requirements or protein synthesis at a given tissue. Rather, only data regarding growth in the whole body are obtained. Another strategy to rate proteins is to compare the AA profile in food protein to some reference protein. Previously, food proteins such as egg or milk were used as a reference but there has been a recent move toward the use of an idealized reference pattern of AAs to rate proteins. This assumes that the true requirements for a given AA are known, which is discussed in section 6. Ultimately, all the methods of rating protein quality described above are insufficient for rating proteins for bodybuilders. They are used primarily to determine minimum requirements to either support optimal growth in children (which differs physiologically from the growth seen in bodybuilders since much of the tissue synthesized is organ, and not muscle tissue) or maintenance in adults. None are meant (or should be) used to determine the quality of various proteins for an adult bodybuilders interested in gaining muscle tissue (who is maintaining other bodily tissues). In part 3 of this series, we will further examine the issue of protein quality by looking at AA requirements. Various dietary proteins will be examined within this context. Part 3 will also include the development of a basic kinetic model of AA flow within the body including the various fates that amino acids may have. This model will be used to examine the adaptations which occur to both high and low protein intakes so that dietary strategies such as protein cycling can be examined.
References
1. National Research Council. Recommended Dietary Allowances, 10th ed. National Academy Press, 1989. 2. Moriarty, KJ et. al. Relative nutritional value of whole protein, hydrolysed protein and free amino acids in man. Gut (1985) 26: 694-699. 3. Young, VR. Soy protein in relation to human protein and amino acid nutrition. J Am Diet Assoc (1991) 91: 828-835. 4. Wagenmakers, AJM. Muscle amion acid metabolism at rest and during exercise: role in human physiology and metabolism. Exercise and Sports Science Reviews (1998) 26: 287-314. 5. Advanced Nutrition and Human Metabolism, 2nd ed. James L. Groff, Sareen S. Gropper, Sara M. Hunt. West Publishing Company, 1995. 6. Energy and protein requirements. Report of a joint FAO/WHO/UN expert consultation. WHO technical report series 724. Geneva: World Health Organizations, 1985. 7. Young, VR and El-Khoury, AE. Can amino acid requirements for nutritional maintenance in adult humans be approximated from the amino acid composition of body mixed proteins? Proc Natl Acad Sci USA (1995) 92: 300-304. 8. Millward, DJ. Metabolic demands for amion acids and the human dietary requirement: Millward and Rivers (1988) revisited. J Nutr (1998) 128: 2563S-2576S. 9. Pellett, PL and Young, VR. Nutritional evaluation of protein foods. United Nations University, 1980. 10. Poullain, MG et. al. Effect of whey proteins, their oligopeptide hydrosylates and free amino acid mixtures on growth and nitrogen retention in fed and starved rats. J Parenteral and Enteral Nutrition (1989) 13: 382-386. 11. Normal and Therapeutic Nutrition, 17th ed. Corinne H. Robinson, Marilyn R. Lawler, Wanda L. Chenoweth, and Anne E. Garwick. Macmillan Publishing Company, 1986. 12. Henley, EC. Food and Drug Administration’s proposed labeling rules for protein. J Am Diet Assoc (1992) 92: 293-294, 296. 13. Young, VR. and Pellett, PL. Protein evaulation, amino acid scoring and the Food and Drug Administrations’s proposed food labeling regulations. J Nutr (1991) 121:145-150. 14. Hernandez, M et. al. The protein efficiency ratio of 30:70 mixtures of animal:vegetabls protein are similar or higher than those of the animal foods alone. J Nutr (1996) 126: 574-581. 15. Food and Agriculture Organization and World Health Organization (1990) protein quality evaluation. Report of a joint FAO/WHO expert consultation. Food and agriculture organization of the United Nations, Rome, Italy. 16. Young, VR and Pellett, PL. Plant proteins in relation to human protein and amino acid requirements. Am J Clin Nutr (1994) 59 (suppl): 1203S-1212S.
About the author
Lyle McDonald+ is the author of the Ketogenic Diet as well as the Rapid Fat Loss Handbook and the Guide to Flexible Dieting. He has been interested in all aspects of human performance physiology since becoming involved in competitive sports as a teenager. Pursuing a degree in Physiological Sciences from UCLA, he has devoted nearly 20 years of his life to studying human physiology and the science, art and practice of human performance, muscle gain, fat loss and body recomposition.
Leave a Reply
You must be logged in to post a comment.