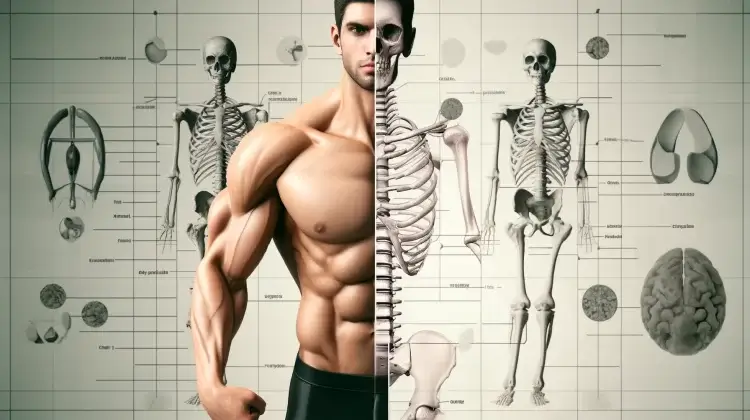
Estimated reading time: 19 minutes
Table of contents
Introduction
Anabolic-Androgenic Steroids (AAS) and Recombinant human Growth Hormone (RhGH) Effects on Bones, Tendons, Ligaments and Joints, and a Per-Compound Assessment
Collagen is the primary structural component of all connective tissue (primarily type I collagen for bone, tendon, and ligaments; and primarily type II collagen for cartilage). Type III collagen activity reflects soft tissue growth and turnover, it is widely distributed in the interstitial fibrils of soft tissue including tendon and ligaments, fascia, as well as the extracellular matrix (ECM), and its layers (e.g., endomysium) that envelop the constituent parts of muscle groups and that contain various growth factors involved in skeletal muscle function and growth.
Collagen metabolism encompasses the biochemical processes that regulate synthesis, breakdown, and turnover of soft tissues that are comprised primarily of collagen. Our body’s connective tissues are in a constant state of equilibrium and flux. Collagen metabolism maintains the structural integrity and function of the body’s joints, bones, tendons, and ligaments. Enzymes and signaling pathways regulate these processes of synthesis, breakdown, and turnover.
Procollagen, the parent protein to collagen, is synthesized and secreted by fibroblasts. Procollagen molecules consist of 3 protein strands arranged in a triple helix. Procollagen leaves the cell with protective extensions on the ends to prevent premature collagen formation. Cleavage of the extensions via enzymes results in the formation of active collagen that aligns with other collagen molecules.
Key Point: Measurement of these enzymes (i.e., markers) provides an indication of collagen metabolism. An increase or in certain enzyme levels indicates net (e.g., type I) collagen synthesis, and a decrease or increase in certain enzyme levels indicates net (e.g., type I) collagen breakdown. |
A microfibril is a unit of collagen filaments arranged into parallel. These are the subunit of collagen fibers. Fibers are arranged into bundles. Cross-linking between adjacent collagen molecules throughout the collagen bundles are strong chemical bonds that provide integrity and a robust lattice of connective tissues that support the skeleton in locomotion.
Collagen Type I is particularly important for changes to bone mineral content and bone mineral density (BMC/BMD).
Collagen Type III is particularly important for changes in ECM, tendon, ligaments, and:
- Force transmission from sarcomere to bone (increased strength)
- Recovery from musculoskeletal injury, particularly involving the collagen matrix
- Prevention of overuse or acute musculoskeletal injury (by increasing force-to-failure).
Generally AAS affect collagen metabolism. Supraphysiological AAS:
- ↑ serum PIIINP [dose-dependently]
- ↑ urine HP:LP
- High AAS doses enhance soft tissue collagen metabolism, with no change in bone resorption. [1].
Withdrawal (“cycling-off”) from AAS:
- ↓ serum ICTP [time-dependently]. [1].
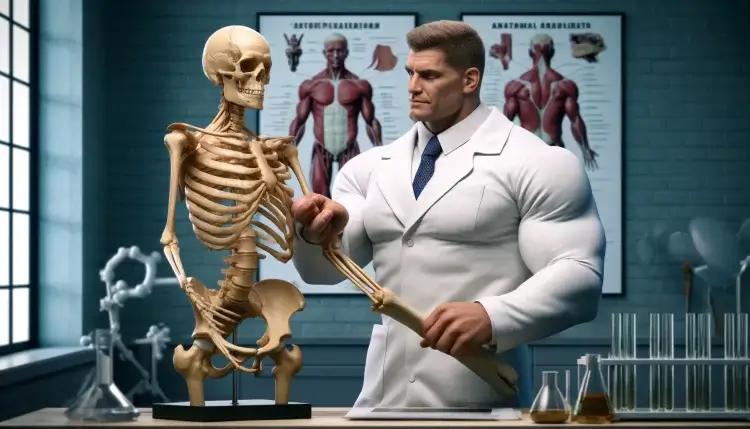
Markers of Collagen Metabolism
Tendon (Collagen Metabolic Markers)
Procollagen Type III N-terminal propeptide (PIIINP) is a marker of interstitial fibril biosynthesis in soft tissues. PIINP is stimulated by plyometric training in tendon as well as by AAS generally, where stiffness benefits velocity, but muscular strength gains must compensate for the heightened risk of muscular strain due to excessive tendon stiffness.
- Increased by supraphysiologic AAS dose-dependently.
Bone and Tendon (Collagen Metabolic Markers)
Procollagen Type I C-terminal propeptide (PICP): released into the circulation by proliferating osteoblasts during collagen biosynthesis, and is therefore largely a biomarker of bone collagen formation, although there will also be some contribution from type I collagen in soft tissues. [2].
Crosslinked telopeptide of Type I collagen (ICTP): released into circulation during the osteoclastic phase of bone modeling and remodeling (bone/collagen breakdown). [2].
- Decreased by withdrawal from supraphysiologic AAS (“cycling-off”) time-dependently.
Dpyr: urinary marker of type I collagen breakdown. [2].
Both ICTP and Dpyr constitute pyridinoline crosslinks formed in mature collagen type I, and their concentrations in serum and urine reflect bone resorption. [2].
Bone (Collagen Metabolic Markers)
Bone alkaline phosphatase (ALP): a protein found in bone cells of in the epiphyseal growth plate and in mature osteoblasts. This is irrelevant for muscle size or strength. [2].
HP (PYD): hydroxylysylpyridinoline; reflects turnover of collagens type I (bone), II, III and IX; present in tendon, cartilage, bone, vessel walls and dentine. [2].
LP (DPD): lysylpyridinoline; reflects turnover of collagen type I (bone); present in bone and dentine.
There is an age-associated decline in HP (↓), LP (↓), and HP/LP ratio (↓).
HP:LP (urine) excludes skin metabolism (useful). [2].
The urinary HP and LP are potentially more useful markers of the catabolism of collagen fibres from skeletal tissues than urinary hydroxyproline. The latter has a lower specificity profile since it is found in all collagen types of all connective tissues (including skin). Moreover, it may also be released from collagen molecules prior to their incorporation into fibrils, and a large proportion of hydroxyproline is metabolized in the liver, thereby evading quantitative analysis of the resorption of mature collagen by urinary measurements. [3].
Lower HP:LP may reflect higher relative proportion of bone resorption vs. collagen and cartilage turnover since bone collagen is the primary LP source whereas HP reflects soft tissues generally (except skin)… suggests that resorption of type III collagen (more HP than type I) also decreases with age. [3].
- Increased by supraphysiologic AAS.
Peak bone mass and HP:LP occurs at age 27. [3].
Bone
Bone Cells
Osteoprogenitors: Osteogenic cells that develop into osteoblasts.
Osteoblasts: Bone forming; produce bone ECM and mineralize bone (mononucleate).
Osteocytes: Mature bone cell; enzyme-secreting to maintain bone.
Osteoclasts: Bone degrading (resportion of aged bone).
Cell type | Function | Location |
Osteoprogenitor | Develop into osteoblasts | Deep layers of the periosteum and the marrow |
Osteoblast | Bone formation | Growing portions of bone, including periosteum and endosteum |
Osteocyte | Maintain mineral concentrations of matrix | Entrapped in matrix |
Osteoclast | Bone resorption | Bone surfaces and at sites of old, injured, or unneeded bone |
Bone Metabolism
Osteoblastic bone formation is associated with deposition of type I collagen, followed by mineralization and maturation during which stable cross-links are formed between collagen fibrils. [4].
Osteocalcin (serum): Reflects bone formation (as well a procollagen type I [serum]).
Deoxypiridinoline (urine): Reflects bone resorption. [4].
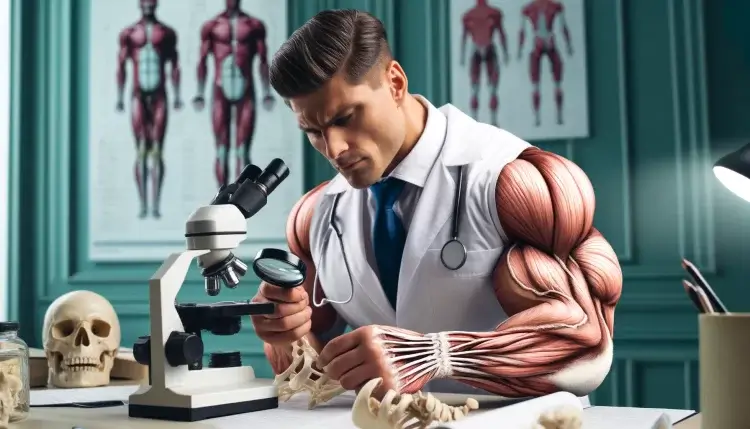
Tendon and Ligament
Tendon is connective tissue connecting bone to muscle, whereas ligament is connective tissue connecting bone to bone. In both, roughly ¾ of dry weight is collagen: most is type I: 60% (tendon) and up to 85% (ligament).
The relatively (almost totally) avascular, collagenous structure of ligaments and tendons limits their regenerative potential, resulting in substantial medical complications, often necessitating surgery after traumatic injury.
Mature tendon and ligaments contain relatively few cells. The small number of metabolically-active cells therein ↓O₂ and nutrient requirements. Ligaments contain elastin fibers and collagen.
The mechanical properties of tendon and ligament are a function of:
- Collagen fiber density
- Diameter
- Orientation and
- Cross-linking
- Enzymatic cross-links, formed by lysyl oxide (LOX)
- Non-enzymatic cross-links through AGEs (advanced glycation end-products), formed by a Maillard reaction w/o specific enzyme between a sugar + amino acid.
- Both cross-links increase the stiffness of tendon and ligament. [5].
Training Adaptations
Tendon metabolism is much slower than muscle metabolism due to its reduced vascularity and circulation, and the increased blood flow to skeletal muscle via exercise is not paralleled by the same flow perfusion in tendon. [6].
Muscle hypertrophy relates to an ↑ in # and size of fibroblasts, thereby ↑ total collagen supply. Fibroblast activation and subsequent connective tissue growth are prerequisites for hypertrophy [7], such that collagen content is maintained in proportion to muscle mass.
Tendon stiffness refers to force transmission per unit of strain, or tendon elongation. Increased stiffness of ligament a Good Thing (✓), as stiffness is beneficial to maintain joint stability and ↓injury risk. Conversely, since tendon connects stiff bone to compliant muscle, a stiffer tendon is not always beneficial:
- In terms of performance: ↑stiffness (tendon) transmits muscle forces faster to bone, thereby ↑performance; However,
- this interest must be balanced against the potential for strain concentration within the muscle:
- More strain (“stretch”) produced in a given movement is concentrated in the muscle that is connected to a stiff tendon ⇒ isometric contraction rather than tendon stretching pliably while muscle contracts.
A stiff tendon does not stretch; rather, it is forced to lengthen while contracting (eccentric), therefore a muscle attached to a stiff tendon experiences greater eccentric load for a given movement has an increased injury risk. [5].
Androgen Effects on Tendon
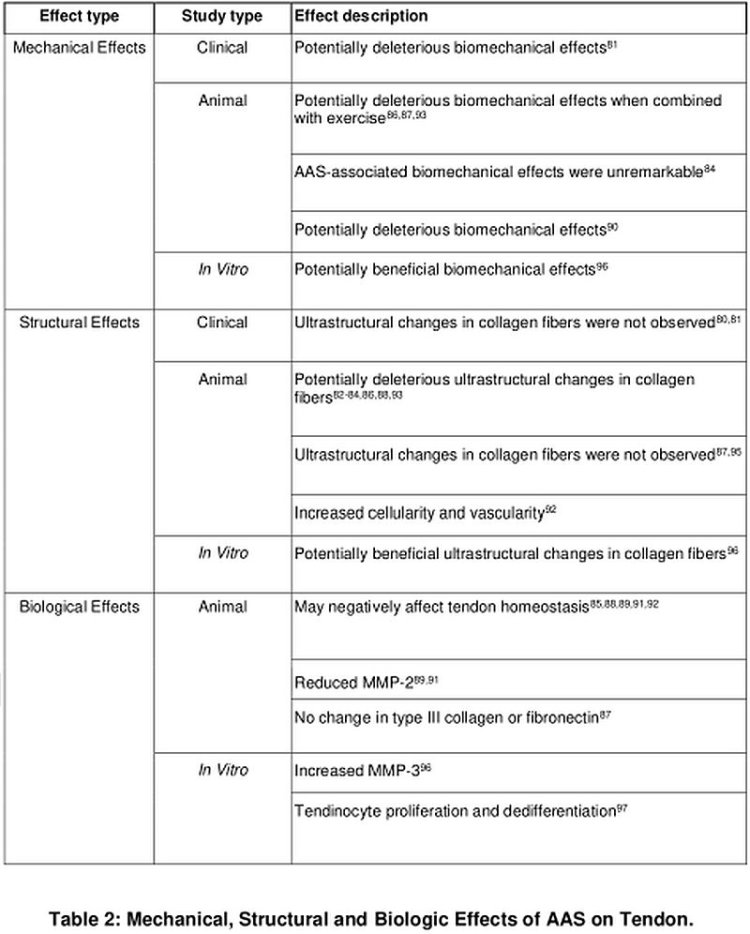
The upshot from this Table shows that the effects of AAS on tendon, and more importantly the muscle-tendon unit, are not conducive to a soundbyte description or straightforward catch-all conclusion, but are nuanced due to heterogeneity of the data. AAS variously enhance and hinder different elements of tendon structure and function.
In summary, practical conclusions to be drawn from the literature about AAS effects on tendon are that:
- The potentially deleterious biomechanical effects of AAS may be transient.
- Systemic and locally administered AAS may affect tendon similarly.
- Considerable gaps in knowledge exist with respect to:
- Tendon injury/pathology
- Dose-response
- Drug-response (most studies use Nandrolone; some Metandienone)
- Stacking
- Timing, and
- Response population (sex-, age- related differences).
- Tissue-Specific Effects:
- AAS are likely to affect tendon, muscle, and the fibrocartilage metabolism (net synthesis/degradation) differently, but no studies to date have been conducted to characterize these differences. [9].
Joints
The body’s joints are the points at which bones meet (articulation) and that provide for human locomotion (muscles pull bones about joints to move the body). Joints are comprised of bone, tendon (attaching bone to muscle), and ligaments (attaching bone to bone). Classification of joints under various schema is possible (e.g., functional, structural). This article will focus on the body’s synovial joints – that include the hip, knee, shoulder, and elbow. In addition to being comprised of bone, tendon, and ligament, these joints contain synovial cells, a type of cell that contains a lining membrane that produces synovial fluid. Synovial fluid lubricates and nourishes the joint, reduces friction, and provides cushioning between the articulating surfaces. Importantly, this lubrication and nourishment depends on the production of proteins and growth factors.
The effects of AAS on joints are not comprehensively described, but there are per-compound studies that will be reviewed next for insights into particular AAS and their effects on joints. The potential mechanisms by which AAS affect joints relate broadly to effects on collagen metabolism, turnover, breakdown, and synthesis; effects on C1-INH; and synovial joint function.
C1-INH
C1-esterase inhibitor; SERPING1
C1-INH is a multi-serine protease inhibitor that controls several catalytic pathways including classical component activation.
The attenuated androgens – AAS that possess relatively diminished androgenic potency – discussed herein, Oxandrolone and Stanozolol, both 17AAs, induce intrinsic production of C1-INH and ↑catabolism (i.e., breakdown) of bradykinin.
Bradykinin, via its action at B₂R, mediates vasodilation and enhances permeability resulting in angioedema. Hereditary angioedema (HAE), by extension, results from ↓C1-INH. HAE is a condition attenuated androgens may be used to treat.
When C1-INH is decreased, vascular permeability (subcutaneous and submucosal) ensues (“angioedema”) due to ↑bradykinin (which C1-INH attenuates) [due to effects on classical contact system and complement activation that are beyond the scope of this article]. [10].
Androgens upregulate C1-INH gene expression and plasma aminopeptidase P (catabolizes kinins) (55, 56). [10].
Attenuated (17AA) androgens most potently enhance hepatic C1-INH production [23] by direct hepatic action rather than AR action per se ⇒ ↑C1-INH and C4 (due to reversal of secondarily depressed C4 levels). [11].
C1/C1-INH complexes are formed when C1-INH combines with and removes C1r and C1s from activated C1 and these complexes – representative of classical complement pathway activation – are associated with arthritic and rheumatological conditions. [12]. This may contribute to the reputation for Stanozolol in particular causing joint pain.
Key Point: Acquired “angioedema without wheals” may be caused by drugs, ACE inhibitors (in 0.1 – 2.2% of users, higher in Africans) being the most common offenders. ACE is required for bradykinin degradation and its inhibition can cause bradykinin accumulation causing angioedema. Androgens may to some extent suppress angioedema by their effects on C1-INH. A forthcoming article by this author discusses the hazards of promulgating antihypertensive drugs, ACE inhibitors especially, as well as ARB drugs, for healthy AAS-using bodybuilders in the absence of chronic hypertension, and addresses the particular (and apparently controversial) matter of ACE inhibitor-induced diminution (blunting) of hypertrophy. |
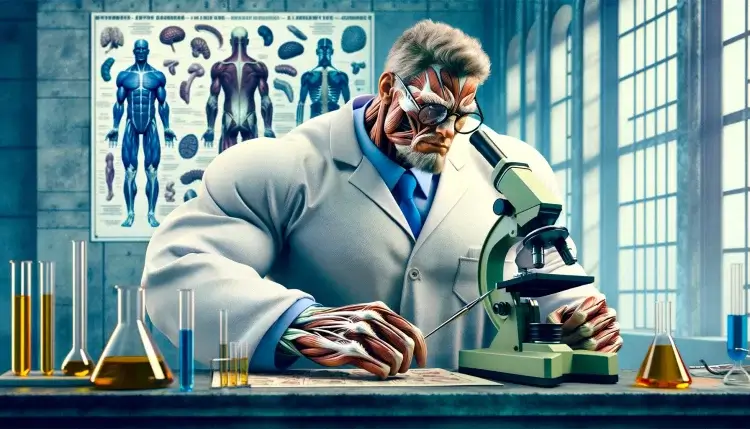
Compounds
Nandrolone
Putative mechanisms that improve joint pain symptoms:
- Increased collagen synthesis and deposition in tendon and ligament
- Synovial joint fluid retention.
Both mechanisms (increased collagen production in, e.g., flexor tendon) and synovial joint fluid retention may be positively acted upon by the renin-angiotensin system (RAS). The RAS regulates water and electrolyte balance, connective tissue cell growth, and the metabolism of loose and dense connective tissue and sites of tissue repair. [13]. Pathologically, RAS activation increases vascoconstriction, cardiac hypertrophy, and fibrosis (resulting in myocardial infarction, fibrosis of the liver). [13]. Therefore, it is important to consider the duality of the potential joint (i.e., knee extensor tendon) augmentation while using nandrolone: you may have a transient benefit in tendon remodeling (i.e., extensor tendon), but via this same mechanism, may be accruing fibrotic or cardiac maladaptations.
So, to the potential transient benefits: Angiotensin-I converting enzyme (ACE) is a marker positively correlated with collagen type I mRNA activity, and may reflect extracellular matrix (ECM) remodeling where collagen synthesis outstrips degradation.
Nandrolone increases ACE activity and increases matrix type I collagen deposition. In a model of training (i.e., plyometric), nandrolone + jump training >> jump training > sedentary with respect to ACE activity in tendon (i.e., knee extensor), suggesting a potential synergy between training and nandrolone in this respect. Consider, however, that this same pathway is implicated in cardiac tissue remodeling and pathological action. Moreover, increased tendon stiffness presents a risk if strength gains do not increase in order to reduce the likelihood of catastrophic injury due to strain concentration. Thus, considerations about training should be planned rigorously, especially if one engages in plyometric training. [13].
Nandrolone’s reputation for enhancing joint function during hard training phases with heavy loads is bolstered by these findings that, by increasing ACE activity, affecting the RAS, it serves to increase net collagen synthesis and fluid balance in the joints.
Oxandrolone
The significantly greater increase to height velocity from treatment with GH plus oxandrolone compared with GH alone was reflected in similar differences in the early response of bone ALP and PICP, both associated with bone formation, but not in PIIINP, a marker of soft tissue turnover, or ICTP, a marker of bone collagen breakdown. This suggests that oxandrolone may, either directly or indirectly, affect osteoblast proliferation and chondrocyte proliferation and maturation – an additive effect to that of GH alone. [2].
The results from Crofton, et al. suggest that GH+oxandrolone > GH+test > GH > placebo in ΔPIINP, yet significant between-group differences may not have been measurable due to small sample size (a potential type 2 error). [2].
The administration of oxandrolone for up to 24 months to severely burned pediatric patients significantly improved whole-body bone mineral content (WB BMC), lumbar spine bone mineral content (LS BMC), lumbar spine bone mineral density (LS BMD), and height velocity. [14].
A severe burn injury induces a hypermetabolic and hyper-catabolic response that is characterized by increases in cardiac work, resting energy expenditure, and muscle protein degradation (1–6). This compensatory response is accompanied by elevated hepatic glucose production and insulin resistance (4, 5, 7–10). Patients typically experience loss of lean body mass, and in children, growth is impeded. Significant reduction of bone mineral content (BMC), bone mineral density (BMD), and adipose tissue occurs over time. [14].
The effects on BMC/BMD became significant in these patients only after longer than one year of continuous treatment. [14]. There was a synergy in this effect modulated by the growth maturation phase in pediatric patients, that may have ramifications for adult use of rhGH and aromatizing androgen, associated with the pubertal surge. The supposition that one might draw is that long-term aromatizing androgen (e.g., T, nandrolone) use in combination with high rhGH doses may mimic some aspects of the growth maturation phase in children, potentially enhancing BMC and BMD synergistically in combination with oxandrolone when used over long time-frames.
Oxandrolone’s particular effects on bone mineral content and density in growing children suggests that it is particularly useful in the early phase (i.e., the first six months) of continuous RhGH use where bone turnover is increased.
Stanozolol
Stanozolol, popularly associated with joint pain (“achy, dry joints”) affects synovial fibroblasts, precursors to cells that comprise the synovial joints (e.g., hip, knees, shoulders), by inhibiting DNA synthesis. [10]. While stanozolol is regarded as particularly potent in stimulating procollagenase activity in scalp, an effect that is associated with increasing TGF-β1 secretion [15] – thereby stimulating procollagenous activity, but perversely by this same mechanism, inducing androgenic alopecia in scalp – the effect on the synovial cell is qualitatively different.
Stanozolol’s inhibiting DNA synthesis in the synovial cell, coupled with its effects on C1-INH, provide several explanatory modes for its causing aching in the synovial joints.
Testosterone
Testosterone (250 mg weekly) caused modest increases in collagen metabolism biomarkers, increasing PINP by 28%, ICTP by 22%, and PIINP by 70%. [16]. Testosterone increased type I (bone; PINP, ICTP) and type III (tendon and ligament, soft tissues; PIINP) and particularly enhanced the effect of rhGH on type III markers (PIINP), suggesting a strong modulating effect of testosterone on the type III collagen comprising tendon, ligament, and likely fascia in response to GH. [16].
RhGH
Administration of rhGH into the tendon or ligament results in a marked increase in collagen metabolism resulting in net deposition. [17]. RhGH increases the activity of osteocalcin and procollagen type I in serum, reflecting augmented BMD/BMC. In skeletal muscle and tendon the extracellular matrix (ECM) confers important tensile properties and is crucially important for tissue regeneration post-injury. [10]. Administration of rhGH promotes ECM collagen synthesis in musculotendinous tissue in healthy young adults.
RhGH increased collagen I (bone) mRNA by 2.3-fold and collagen III (ECM, tendon, ligaments) by 2.5-fold. [10]. A tendency towards a 5.8-fold increase in muscle collagen protein synthesis was observed. [10]. The dosage used was 33.3 µg * kg⁻¹ * day⁻¹ during the first seven days and 50 µg * kg⁻¹ * day⁻¹ in healthy young men thereafter. [10]. This equates to a 3 – 4.5 IU rhGH daily dose for a 90 kg male.
There is a biphasic (two-pronged) change in BMD in response to rhGH administration in the GHD population, with an initial decrease around six months after therapy initiation, followed by a subsequent increase after at least one year of treatment. [18]. The prevailing hypothesis is that GH stimulates both bone formation and bone resorption, which results in increased bone turnover. [18]. This effect is prominent during at least the first six months of rhGH administration, resulting in a decrement in BMD and bone mineral content. [18]. No dose-response relationship has been established due to wide variation in dosages used. [18]. It appears that trabecular (lumbar spine) bone has a different sensitivity versus cortical (femoral neck) bone to GH. [18].
RhGH combines at least additively in some respects with androgen (e.g., oxandrolone, testosterone) and synergistically in others with respect to soft tissue metabolism. RhGH and testosterone, when used in combination, particularly stimulate PIINP, suggesting a synergistic effect on tendon, ligament, and likely fascia.
Conclusion
AAS have class effects, as well as drug-specific effects, on the joints and connective tissues that comprise them. Generally, AAS enhance soft tissue metabolism with no effect on bone resorption, but it is important to consider the various positives and negatives inherent to AAS with respect to tendon.
Aromatizing androgen, typified by testosterone, has synergistic (greater than additive) effects in combination with GH on some aspects of collagen metabolism, particularly in its aspects that relate to soft tissue metabolism (e.g., fascia), and additive effects in other respects.
The nonaromatizable 17AAs oxandrolone (a 5α-androstan-3-one) and stanozolol (an AAS whose A-ring has a pyrazole ring juncture) share certain class effects, e.g., stimulating intrinsic production of C1-INH that is associated with rheumatological and arthritic conditions, but stanozolol stands apart in its demonstrable dose-dependent inhibition DNA synthesis in synovial cells that comprise major body joints, modulating connective tissue breakdown. Oxandrolone has been demonstrated to additively augment bone mineral density (BMD/BMC) and may be particularly useful during the first six months of initiating a course of aromatizing androgen and rhGH to enhance bone mass retention.
Key Point: A nuanced approach to decision-making about training loading (e.g., progression, intensity, mode) and drug effects (e.g., AAS, rhGH) with respect to joints, tendons, ligaments, and bone, is made possible by a close reading of this article. For practitioners, particular areas to read attentively relate to tendon stiffness and implications for those engaging in plyometric or even light-load training; pros and cons of AAS on biological, structural, and mechanical aspects of tendon; and the dearth of strong data pertaining to soft tissue effects by different AAS. |
References
[1] Pärssinen, M., Karila, Kovanen, and Seppälä. (2000). The Effect of Supraphysiological Doses of Anabolic Androgenic Steroids on Collagen Metabolism. International Journal of Sports Medicine, 21(6), 406–411. doi:10.1055/s-2000-3834
[2] Crofton, P. M., Stirling, H. F., Schönau, E., and Kelnar, C. J. H. (1996). Bone alkaline phosphatase and collagen markers as early predictors of height velocity response to growth-promoting treatments in short normal children. Clinical Endocrinology, 44(4), 385–394. doi:10.1046/j.1365-2265.1996.706cn527.x
[3] Açil, Y., Brinckmann, J., Notbohm, H., Müller, P. K., and Bätge, B. (1996). Changes with age in the urinary excretion of hydroxylysylpyridinoline (HP) and lysylpyridinoline (LP). Scandinavian Journal of Clinical and Laboratory Investigation, 56(3), 275–283. doi:10.3109/00365519609088617
[4] Aerssens, J., Van Audekercke, R., Geusens, P., Schot, L. P. C., Osman, A. A.-H., and Dequeker, J. (1993). Mechanical properties, bone mineral content, and bone composition (collagen, osteocalcin, IGF-I) of the rat femur: Influence of ovariectomy and nandrolone decanoate (anabolic steroid) treatment. Calcified Tissue International, 53(4), 269–277. doi:10.1007/bf01320913
[5] Chidi-Ogbolu N, Baar K. Effect of Estrogen on Musculoskeletal Performance and Injury Risk. Front Physiol. 2019;9:1834. Published 2019 Jan 15. doi:10.3389/fphys.2018.01834
[6] Kjaer, M.J. (2008). Role of extracellular matrix in adaptation of tendon and skeletal muscle to mechanical loading. Physiol. Rev. 84:649-698.
[7] Joblecki, C.K., Heuser, J.E., Kaufman, S. (1973). Autoradiographic localization of new RNA synthesis in hypertrophying skeletal muscles. Journal of Cellular Biology. 57:743-759.
[8] Spadari, A., Romagnoli, N., Predieri, P. G., Borghetti, P., Cantoni, A. M., and Corradi, A. (2013). Effects of intraarticular treatment with stanozolol on synovial membrane and cartilage in an ovine model of osteoarthritis. Research in Veterinary Science, 94(3), 379–387. doi:10.1016/j.rvsc.2012.11.020
[9] Jones, I. A., Togashi, R., Rick Hatch, G. F., Weber, A. E., and Vangsness JR, C. T. (2018). Anabolic Steroids and Tendons: A Review of their Mechanical, Structural and Biologic Effects. Journal of Orthopaedic Research®. doi:10.1002/jor.24116
[10] Ellis AJ, Cawston TE, Mackie EJ. The differential effects of stanozolol on human skin and synovial fibroblasts in vitro: DNA synthesis and receptor binding. Agents Actions. 1994 Mar;41(1-2):37-43. doi: 10.1007/BF01986391. PMID: 8079819.
[11] Wright JK, Smith AJ, Cawston TE, Hazleman BL. The effect of the anabolic steroid, stanozolol, on the production of procollagenase by human synovial and skin fibroblasts in vitro. Agents Actions. 1989 Nov;28(3-4):279-82. doi: 10.1007/BF01967415. PMID: 2556901.
[12] Oleesky DA, Daniels RH, Williams BD, Amos N, Mor gan BP. Terminal complement complexes and C1/C1 inhibitor complexes in rheumatoid arthritis and other arthritic conditions. Clin Exp Immunol. 1991;84(2):250-255.
[13] Marqueti, R. de C., Hashimoto, N. Y., Durigan, J. L. Q., Batista e Silva, L. L., Almeida, J. A. de, Silva, M. da G. da, … Araújo, H. S. S. de. (2015). Nandrolone increases angiotensin-I converting enzyme activity in rats tendons. Revista Brasileira de Medicina Do Esporte, 21(3), 173–177. doi:10.1590/1517-869220152103143667
[14] Reeves, P. T., Herndon, D. N., Tanksley, J.D., Jennings, K., Klein, G. L., Mlcak, R. P., … Finnerty, C. C. (2016). FIVE-YEAR OUTCOMES AFTER LONG-TERM OXANDROLONE ADMINISTRATION IN SEVERELY BURNED CHILDREN. Shock, 45(4), 367–374. doi:10.1097/shk.0000000000000517
[15] Falanga, V., Greenberg, A. S., Zhou, L., Ochoa, S. M., Roberts, A. B., Falabella, A., and Yamaguchi, Y. (1998). Stimulation of Collagen Synthesis by the Anabolic Steroid Stanozolol. Journal of Investigative Dermatology, 111(6), 1193–1197. doi:10.1046/j.1523-1747.1998.00431.x
[16] Nelson, A. E., Meinhardt, U., Hansen, J. L., Walker, I. H., Stone, G., Howe, C. J., … Ho, K. K. (2008). Pharmacodynamics of Growth Hormone Abuse Biomarkers and the Influence of Gender and Testosterone: A Randomized Double-Blind Placebo-Controlled Study in Young Recreational Athletes. The Journal of Clinical Endocrinology and Metabolism, 93(6), 2213–2222.
[17] Vestergaard, P., Jørgensen, J. O. L., Olesen, J. L., Bosnjak, E., Holm, L., Frystyk, J., … Hansen, M. (2012). Local administration of growth hormone stimulates tendon collagen synthesis in elderly men. Journal of Applied Physiology, 113(9), 1432–1438. doi:10.1152/japplphysiol.00816.2012
[18] Barake, M., Klibanski, A., and Tritos, N. A. (2014). Effects of Recombinant Human Growth Hormone Therapy on Bone Mineral Density in Adults With Growth Hormone Deficiency: A Meta-Analysis. The Journal of Clinical Endocrinology and Metabolism, 99(3), 852–860. doi:10.1210/jc.2013-3921
About the author
Type-IIx is a physique coach, author, and researcher. Bolus: A Practical Guide and Reference for recombinant Human Growth Hormone Use will be his first published textbook, anticipated for release in early 2023. Ampouletude.com will be Type-IIx's base of operations for coaching services and publications. Type-IIx is proud to be a contributing writer to MesoRx, his home forum, where he is a regular poster.
1 reply
Loading new replies...
Join the full discussion at the MESO-Rx →