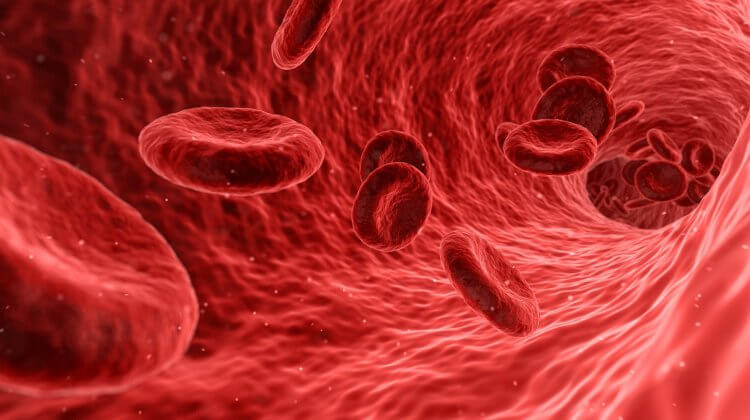
Estimated reading time: 15 minutes
Table of contents
Introduction
The use of anabolic steroids can lead to a condition called erythrocytosis. It’s a condition in which your body has too many erythrocytes, or red blood cells, in its blood. Sometimes this is also referred to as polycythemia. The percentage of blood volume occupied by red blood cells is called hematocrit. For men the reference range is 41 to 51 % and for women the reference range is 36 to 47 %. (There are some slight lab to lab deviations in these reference ranges.)
The red blood cells are responsible for the transport of gases. More specifically, they transport the oxygen from your lungs to the rest of your body. Additionally, they also transport some of the carbon dioxide your body generates back to the lungs. The red blood cells can transport oxygen by virtue of a special protein they contain in large quantities: hemoglobin. Hemoglobin levels can also be measured in the blood, and their reference range spans from 13.5 to 17 g/dL in men and 12 g/dL to 15.5 g/dL in women.
Hematocrit levels and hemoglobin can be easily derived from each other as the two, in general, are very tightly correlated. The hemoglobin concentration in g/dL multiplied by three gives the hematocrit value in %. Thus someone with a hemoglobin level of 15 g/dL has a hematocrit value of about 45%.
When hematocrit gets too low, the body has less capacity to nurture the oxygen needs of its cells. Or in other words: anemia. This can make you feel tired, weak and easily out of breath. This is most pronounced during (strenuous) exercise which requires a lot of oxygen.
When hematocrit gets too high, a different problem arises. The higher it is, the ‘thicker’ your blood becomes: it increases the viscosity of blood. This carries an increased risk of thrombosis. Let’s take a look at how anabolic steroids increase hematocrit and by how much. After that, let’s look at the risk of thrombosis and what might be done about it.
How do anabolic steroids cause erythrocytosis?
Two main factors seem involved in anabolic steroid-induced erythrocytosis. One is an increase in erythropoietin (EPO). A hormone that sometimes makes the news, as it’s used as doping to enhance the performance in endurance sports such as cycling.
EPO is produced in the kidneys in response to reduced oxygen availability. EPO then tells the bone marrow, where the red blood cells are produced in a process called erythropoiesis, to increase red blood cell production. The increase in red blood cells increases oxygen availability and as a result lowers EPO again. Thus there’s a tight balance between hematocrit and EPO.
The other factor that seems involved is suppression of a protein called hepcidin. Hepcidin is a key regulator of iron metabolism, as it regulates the amount of iron that’s absorbed by the gastrointestinal tract [1]. Iron is a pivotal part of hemoglobin: it’s responsible for the binding with oxygen. Suppression of hepcidin leads to an increase in iron uptake, whereas an increase in hepcidin decreases iron uptake. With inadequate iron intake, one would develop anemia. Conversely, iron overload (hemochromatosis) appears to predispose someone to increased hemoglobin/hematocrit levels. Indeed, in (hereditary) hemochromatosis these levels can be elevated [2, 3].
While testosterone administration for 20 weeks shows a dose-dependent increase (up to 600 mg testosterone enanthate weekly) in hemoglobin & hematocrit, especially in older men, it did not in EPO [4]. In contrast, testosterone administration led to suppression of serum hepcidin in young and older men [5]. The testosterone dosage (up to 600 mg enanthate weekly) was highly correlated with the amplitude of this suppression. The increase in hematocrit as a result of testosterone administration is most pronounced in older men. Notably, this study found that the greater increases in hematocrit in older men during testosterone therapy were related to a greater suppression of hepcidin. The authors also measured hepcidin at multiple points in time: at baseline, week 1, week 2, 4, 8 and 20. As such, they could see how it evolved over time. In the first couple of weeks, there was a strong decrease, after which hepcidin levels increased back towards baseline levels—albeit still lower than first.
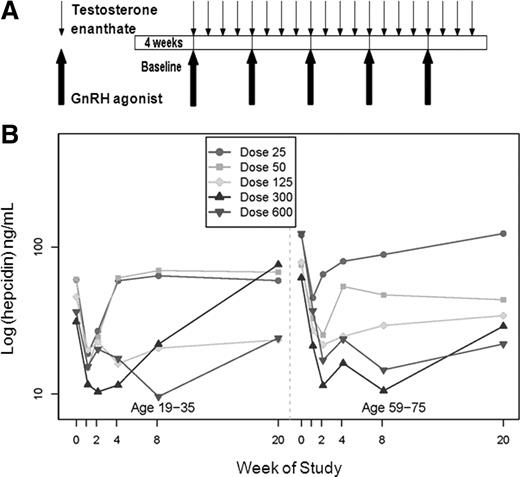
This was one issue with the study which demonstrated no change in EPO after 20 weeks [4]. Perhaps EPO increased initially, but were back to baseline at the 20-week mark. As such, an initial effect would’ve been missed. Additionally, EPO levels demonstrate a wide variability, making it hard to find statistically significant changes, as a relatively large sample size is required.
A future study measured EPO and hepcidin at various points in time in older men with low testosterone receiving either a placebo or testosterone for 6 months [6]. They measured these values at baseline, 1 month, 3 months, 6 months, and 9 months (so 3 months after cessation of the placebo or testosterone). Their results demonstrated an initial increase in EPO in response to the testosterone gel compared to placebo. After, it slowly dropped down showing no difference anymore from placebo at the 6-month mark:
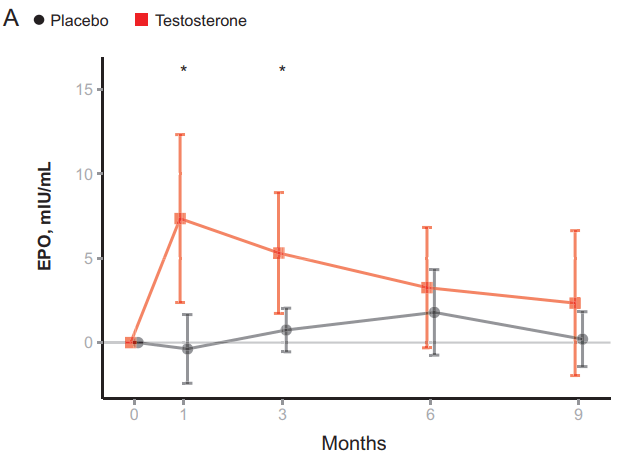
A similar, but inverse, change could be found for hepcidin:
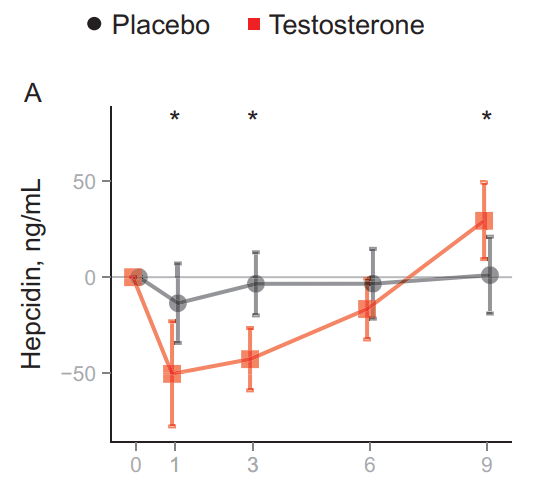
This led the authors to propose that testosterone administration establishes a new EPO/hemoglobin set point. Normally, when hematocrit increases, EPO will decrease in response. In this situation, however, hematocrit increases, most likely, as a result of the increased EPO and decreased hepcidin concentrations. However, once the full increase in hematocrit is established, EPO levels remain similar to what they were before, instead of being decreased. In other words: a new setpoint is established. This is illustrated by the authors in the following figure:
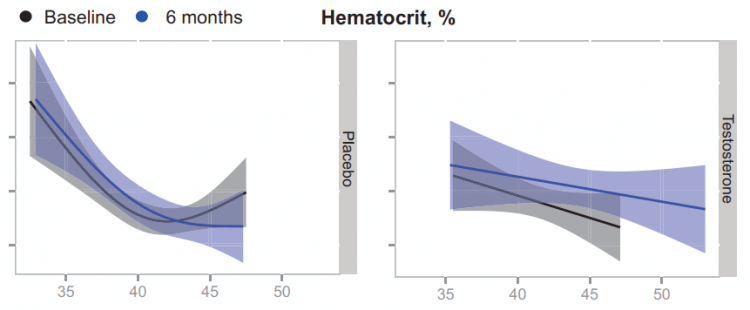
In summary, androgens increase hematocrit/hemoglobin through an initial rise in EPO levels and concurrent decrease in hepcidin levels, which then gradually fall back to baseline levels in the face of increased hematocrit/hemoglobin: a new EPO/hemoglobin set point. The mechanisms of action responsible, and their relative contributions to this, remain to be established.
By how much do anabolic steroids increase hematocrit/hemoglobin?
In a dose-response trial, with dosages up to 600 mg testosterone enanthate weekly, hemoglobin showed a 1.42 g/dL increase in young men after 20 weeks [7]. This translates to slightly over a 4% point increase in hematocrit. The increase is larger in older men, who show a 2.94 g/dL increase in hemoglobin in response to the same dosage for the same period of time [8].
So what about higher dosages? Luckily, there appears to be a limit to the extent with which AAS can increase hematocrit. Let’s have a look at the HAARLEM trial. The HAARLEM trial was a prospective study conducted by the outpatient clinic for anabolic steroid users in the Netherlands [9]. In brief, 100 anabolic steroid users were followed over time while they self-administered AAS. Mean dosage, based on label information, was 898 mg per week, thus making their AAS cycle quite representative of common usage by bodybuilders. Measurements were taken before, during, as well as 3 months after the end of their cycle and 1 year after the start of their cycle. The researchers saw a 3% point increase in hematocrit of the study subjects at the end of their cycle. (The data hasn’t been published yet as I’m writing this. These findings are through personal communication with DL Smit.) This is in line with the 4% increase seen in the trial in young men. The lead author let me know that the increase in hematocrit seemed to level off at an androgen dosage of about 500 mg weekly. Finally, I’d like to add to this that the study subjects did not perform blood donations, so this was not a confounding factor.
Of course, there’s some variation in these results. Some respond to AAS with larger increases in hematocrit than others. Nevertheless, very high levels of hematocrit seem to be rare as can be seen in the box and whisker plots of the study participants of the HAARLEM trial (T0 = right before the AAS cycle, T1 = at the end, T2 = 3 months after cessation of usage, T3 = 1 year after start of the cycle):
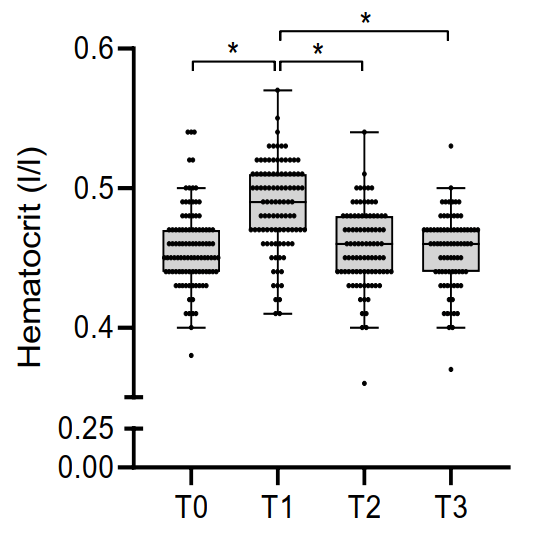
Increased hematocrit is a risk factor for thrombosis
As mentioned earlier, a higher hematocrit makes the blood more viscous. As such, it increases the risk of thrombotic events. This is most pronounced in patients suffering from polycythemia vera. A disease in which the bone marrow overproduces red blood cells, and commonly also white blood cells and platelets (thrombocytes). Median hemoglobin concentration encountered in a large cohort of patients with polycythemia vera was 57% (in men) [10]. On rare occasions, these patients can have hematocrit values over 70%. The highest recorded in this cohort was a whopping 78%. An arterial thrombotic complication was noted in 18% of these patients prior or at diagnosis, and venous thrombosis in 5.4%. (Median age was 59 years.) This is pretty damn high, as the incidence rate of venous thrombosis is about 1 per 1.000 person-years in the age group 55-59 [11]. The risk of venous thrombosis is simply quite low earlier in life, but starts to increase substantially after the age of 50-60, with a peak incidence of 7.9 per 1.000 person-years in the age group 80-84. Leading up to a lifetime risk of 8%.
Of course, looking at polycythemia vera patients isn’t a fair comparison: they also commonly overproduce white blood cells and thrombocytes, as mentioned.
Let’s have a look at the Norwegian Tromsø study [12]. They followed 26,108 subjects over time (median follow-up of 12.5 years) to relate venous thromboembolic events to hematocrit levels. They corrected for age, body mass index and smoking. Obviously there are other factors which could have impacted their results and aren’t corrected for, but at least this corrects for some important confounders. The study showed that for every 5% increase in hematocrit, there was a 33% higher chance of a venous thromboembolic event (in men).
To put this increased risk into perspective: the researchers found about 1.6 venous thromboembolic events per 1.000 person-years in men with an average hematocrit (43-46%). So say you have an average hematocrit level, there would be a 1.6% chance of a thromboembolic event in the next 10 years. If your hematocrit would be 5% higher, i.e. 48-51%, this risk would increase by 33%: 2.1%. This, of course, doesn’t scale linearly forever, but this gives, I believe, a good indication of the increased risk of venous thrombosis as a result of the AAS-induced increase in hematocrit.
Another Danish study found a 26% higher risk of venous thromboembolism in men with a hematocrit above 48% compared to those with a hematocrit between 41.1 and 45% [13]. However, this wasn’t statistically significant. The authors also report risks for arterial thrombosis in the brain and heart. There was a non-statistically significant increase of 27% in the first, and a statistically significant increase of 46% in the latter, when comparing men with a hematocrit above 48% to those with a hematocrit between 41.1 and 45%. (Incidence rates for arterial thrombosis in the brain and in the heart are comparable to those of venous thromboembolism.)
I guess the important part here is that, while there is a substantial increase in relative risk, the absolute risk remains low. This holds especially true for those of young age (younger than 40) and in the absence of other risk factors (i.e. a previous thrombotic event or a coagulation disorder such as factor V Leiden). Whether or not something should be done to counteract the increased risk of thrombosis as a result of the AAS-induced increase in hematocrit therefore seemingly largely depends on the presence of other risk factors. Although one could argue that, in a sense, anabolic steroid use in and on itself is a risk factor. After all, it has a negative impact on more than just hematocrit.
Treatment
Perhaps the best treatment is to lower your dosage substantially (well below 500 mg weekly), or to stop AAS use altogether. This will lower your hematocrit, with its full effect reached after a couple of months, and thus negate the risk. However, this is likely not the most welcomed method to counteract this issue.
A practice I commonly see performed is that of taking a low dosage of aspirin (acetylsalicylic acid). While it doesn’t affect hematocrit levels, it’s widely used for the prevention of cardiovascular diseases [14]. More specifically, it’s used in the secondary prevention of cardiovascular disease. It prevents the clotting of blood by inhibiting an enzyme called cyclooxygenase (COX) in thrombocytes. While this decreases the risk of thrombosis, it also increases the risk of bleeding. And, of course, not just the bleeding of your finger when you cut it while you were working your chicken. But also internal bleeding, such as a hemorrhagic stroke. The benefits should therefore carefully be weighed against the risks of its use. As of current, the European Guidelines on cardiovascular disease prevention in clinical practice thus discourage its use in primary prevention (although this might change for certain populations, such as diabetics) [15].
A common dosage for this purpose is 81 or 100 mg daily. It’s hard to make a general recommendation for its use, although an argument can be made for those at an increased risk (yet perhaps it’s wise to stop AAS use altogether if you do).
As a final note, there’s an increased risk for thrombotic events in the weeks after discontinuation of the drug [16, 17]. Cycling it on and off frequently thus also seems ill-advised.
Phlebotomy (bloodletting) is a way to effectively decrease your hematocrit. One way of doing this is by means of blood donation to a blood bank. However, many countries (and rightfully so) limit the number of times you can do this annually. In my country it’s limited to five times per year. This might not be enough to keep it in the desired range, as one study found persistently elevated hemoglobin levels at repeated visits in a high number of TRT patients that donated blood [18]. If blood donation doesn’t cut it, you can always consult with a general practitioner to perform therapeutic phlebotomy at more frequent intervals.
Keep in mind that you lose iron with each blood donation. As a result, you run the risk of eventually depleting your iron stores and consequently your hemoglobin will remain very low too and you’ll become anemic. It’s possible to counteract this by supplementing iron, but this also drastically cuts the time your body requires to recover the hemoglobin/hematocrit levels [19]. (A dosage of 37.5 mg elemental iron daily was used in the study.) Thus at more frequent intervals, supervision with blood work is advised.
References
- Rauf, Abdur, et al. “Hepcidin, an overview of biochemical and clinical properties.” Steroids 160 (2020): 108661.
- Barton, James C., Luigi F. Bertoli, and Barry E. Rothenberg. “Peripheral blood erythrocyte parameters in hemochromatosis: evidence for increased erythrocyte hemoglobin content.” Journal of Laboratory and Clinical Medicine 135.1 (2000): 96-104.
- Khan, Adnan Aman, et al. “Polycythemia and Anemia in Hereditary Hemochromatosis.” Cureus 12.4 (2020).
- Coviello, Andrea D., et al. “Effects of graded doses of testosterone on erythropoiesis in healthy young and older men.” The Journal of Clinical Endocrinology & Metabolism 93.3 (2008): 914-919.
- Bachman, Eric, et al. “Testosterone suppresses hepcidin in men: a potential mechanism for testosterone-induced erythrocytosis.” The Journal of Clinical Endocrinology & Metabolism 95.10 (2010): 4743-4747.
- Bachman, Eric, et al. “Testosterone induces erythrocytosis via increased erythropoietin and suppressed hepcidin: evidence for a new erythropoietin/hemoglobin set point.” Journals of Gerontology Series A: Biomedical Sciences and Medical Sciences 69.6 (2014): 725-735.
- Bhasin, Shalender, et al. “Testosterone dose-response relationships in healthy young men.” American Journal of Physiology-Endocrinology And Metabolism (2001).
- Bhasin, Shalender, et al. “Older men are as responsive as young men to the anabolic effects of graded doses of testosterone on the skeletal muscle.” The Journal of Clinical Endocrinology & Metabolism 90.2 (2005): 678-688.
- Smit, Diederik L., et al. “Baseline characteristics of the HAARLEM study: 100 male amateur athletes using anabolic androgenic steroids.” Scandinavian journal of medicine & science in sports 30.3 (2020): 531-539.
- Tefferi, Ayalew, et al. “Survival and prognosis among 1545 patients with contemporary polycythemia vera: an international study.” Leukemia 27.9 (2013): 1874-1881.
- Bell, Elizabeth J., et al. “Lifetime risk of venous thromboembolism in two cohort studies.” The American journal of medicine 129.3 (2016): 339-e19.
- Brækkan, Sigrid K., et al. “Hematocrit and risk of venous thromboembolism in a general population. The Tromsø study.” haematologica 95.2 (2010): 270.
- Warny, Marie, et al. “Arterial and venous thrombosis by high platelet count and high hematocrit: 108 521 individuals from the Copenhagen General Population Study.” Journal of Thrombosis and Haemostasis 17.11 (2019): 1898-1911.
- Baigent, Colin, et al. “Aspirin in the primary and secondary prevention of vascular disease: collaborative meta-analysis of individual participant data from randomised trials.” Lancet 373.9678 (2009): 1849-1860.
- F. Hobbs, M. Piepoli, A. Hoes, S. Agewall, C. Albus, C. Brotons, A. Catapano, M. Cooney, U. Corra, B. Cosyns, et al. 2016 european guidelines on cardiovascular disease prevention in clinical practice. European Heart Journal, 37(29):2315–2381, 2016.
- M. Lordkipanidzé, J. G. Diodati, and C. Pharand. Possibility of a rebound phenomenon following antiplatelet therapy withdrawal: a look at the clinical and pharmacological evidence. Pharmacology & therapeutics, 123(2):178–186, 2009.
- L. A. G. Rodríguez, L. C. Soriano, C. Hill, and S. Johansson. Increased risk of stroke after discontinuation of acetylsalicylic acid a uk primary care study. Neurology, pages WNL–0b013e31820d62b5, 2011
- B. Chin-Yee, A. Lazo-Langner, T. Butler-Foster, C. Hsia, and I. Chin-Yee. Blood donation and testosterone replacement therapy. Transfusion, 57(3):578–581, 2017
- Kiss, Joseph E., et al. “Oral iron supplementation after blood donation: a randomized clinical trial.” Jama 313.6 (2015): 575-583.
Image by Arek Socha from Pixabay
About the author
Peter Bond is a scientific author with publications on anabolic steroids, the regulation of an important molecular pathway of muscle growth (mTORC1), and the dietary supplement phosphatidic acid. He is the author of several books in Dutch and English, including Book on Steroids and Bond's Dietary Supplements.
24 replies
Loading new replies...
Join the full discussion at the MESO-Rx →