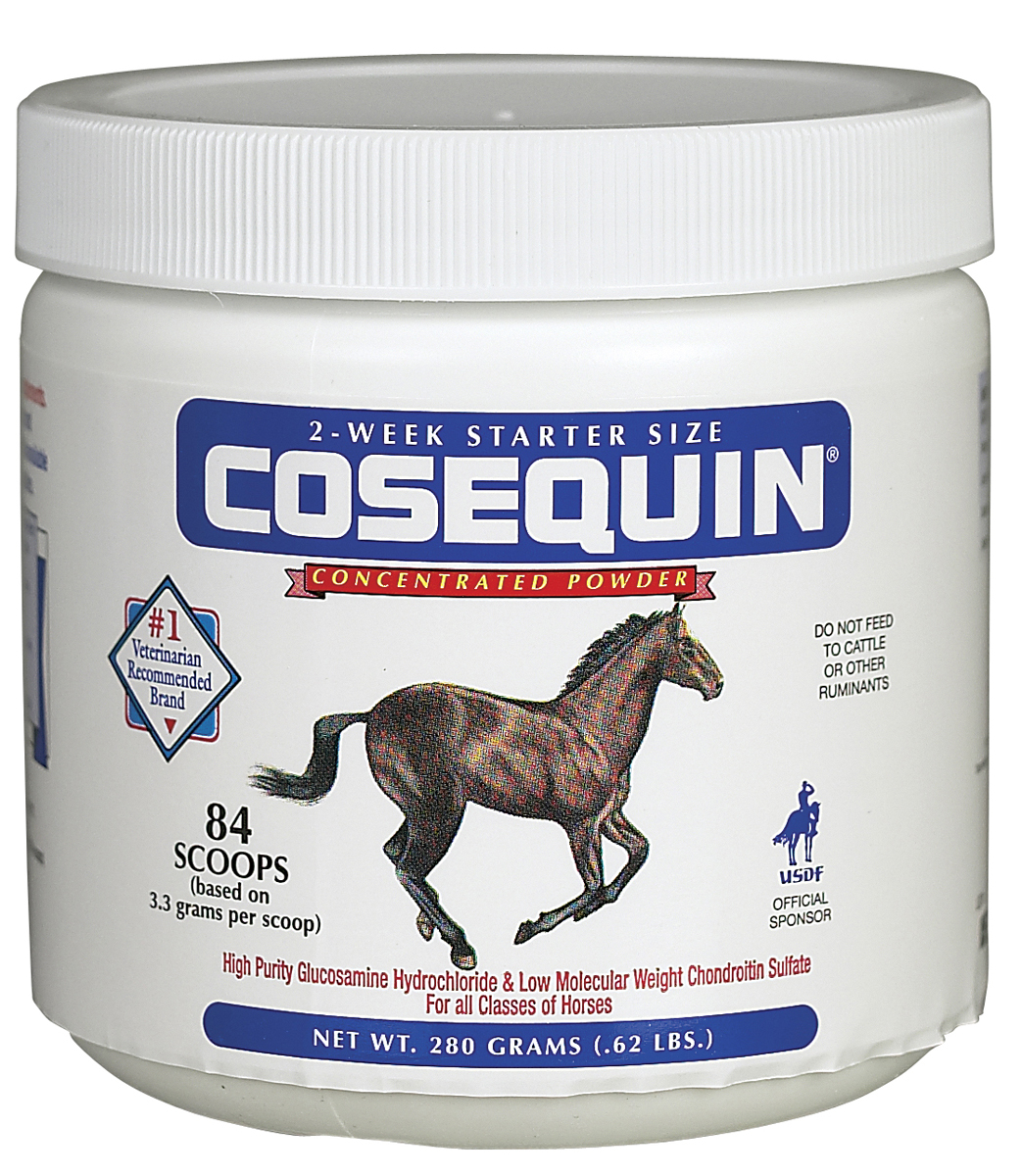
As was discussed in Part II of this series, the major impact of diet upon connective tissue integrity is a deficiency in energy intake, usually associated with inadequate protein and carbohydrates. Aside from its role in mediation of inflammation, there is little research in effects of dietary fats on connective tissue. Micronutrients (minerals and vitamins) have many documented roles in cellular function and thus are critical in the wound healing process. Of nearly any population, athletes generally maintain an adequate diet specifically designed to meet the demands of their sport. Most athletes eat a balanced diet that adequately supplies both macro and micronutrients. Therefore, defects in collagen, elastin and proteoglycan metabolism are generally only seen as a result of deficiencies or excess. As well, successful healing of connective tissue injuries will rely on the presence of adequate nutritional stores. The roles of micronutrients in connective tissue injury healing are discussed in Part III of this series.
As voiced in the field of protein balance, is a state of “accommodation” of micronutrient intake satisfactory in wound healing? (1,2) The body adjusts to changes within different ranges of a nutrient intake. In relation to protein, Waterlow (2) stated that “some adaptations may be at the expense of full functional ability.” for instance, a child may survive on an undernourished diet, but with a slower rate of growth and develop into a smaller-sized adult with sub-average muscle mass and physical strength. Such an adjustment has been termed “accommodation” rather than full adaptation with no loss of function (1,3). This then poses the question of the need for pharmacological supplementation of micronutrients during wound healing. During wound healing is there a need for higher than normally recommended levels to obtain micronutrient balance?
There are several ways a micronutrient deficiency can manifest: deficiencies caused by dietary intake, diet-gene interactions, and nutrient-drug interactions. The first is self-explanatory. Secondly, a genetic dysfunction may produce a differential response to a diet that is deficient or marginal in a nutrient. Additionally, a single or multiple mutant genes may result in expression which resembles a nutrient deficiency or toxicity. These are clinically seen as genetic disorders resulting in abnormal metabolism of micronutrients. Thirdly, some studies suggest that connective tissue defects result from drug interaction with micronutrients.
Those vitamins and minerals that are shown to have profound roles in connective tissue metabolism are addressed along with consequences of their deficiencies on connective tissue metabolism and healing. In addition to diet-induced deficiencies, the article examines some of the defects related to connective tissue metabolism resulting from ingesting drugs that interact with one or more micronutrients. Pharmaceuticals and nutraceuticals most commonly used for connective tissue injuries and healing are discussed separately.
Vitamins
Vitamin C
Of all the vitamins, ascorbic acid probably has the most influence on connective tissue metabolism and has been the most studied. The roles of AA in connective tissue metabolism can be quite complex and varied. Recall from Part I of this series that macromolecule turnover is affected by synthesis and intracellular and extracellular degradation. Important mediators of these two processes are enzymes. Ascorbic acid (AA) is a cofactor for many of the enzymatic reactions in synthetic processes. As described in Part I, degradation of collagen outpaces synthesis; new collagen cannot replace losses and results in disease (scurvy).
In connective tissue, AA is involved in several metabolic reactions. Iron is necessary for a variety of enzymatic reactions, and AA protects iron from oxidation. AA preserves the enzyme-iron complex that catalyzes the reaction for intracellular assembly of collagen (6,35,36). Underhydroxylated collagen is unable to fold into a stable triple-helix (see Part I) and therefore is subject to increased intracellular degradation. The turnover rate of collagen is then in negative balance and degradation outpaces the rate of synthesis.
A large focus of research in AA and connective tissue has been related to the pathophysiology of diabetes. Animal and human diabetics appear to be deficient in plasma concentrations of AA which may be connected to associated delays in wound healing be decreased synthesis of collagen and PGs (33,36). AA is structurally similar to glucose and its cellular uptake is mediated by glucose transport mechanisms. Studies have shown that cellular uptake of AA is inhibited by high extracellular concentrations of glucose (in the presence of as well as in the absence of insulin) as seen in diabetes (4,5,33). This inhibition of AA uptake may exacerbate problems associated with AA deficiencies. Thus, increased intake of dietary AA may prevent inhibition induced by high glucose on collagen and proteoglycan synthesis (4,5).
In addition to collagen, the influence of AA extends to proteoglycans (PGs). AA may serve as a cofactor in sulfation reactions in PGs (4,6,35). However, the exact mechanism of AA influence in GAG metabolism is not yet elucidated.
The most commonly known role of AA is as an antioxidant. Although not clearly demonstrated in the literature, AA may protect macromolecules from damage by free radicals by acting as a scavenger. These deleterious metabolites are highly reactive by-products produced endogenously or by metabolism of drugs in the body. Part II of this series discussed oxidative products produced from metabolism of glucose, one of which is free radicals. Thus, some authors postulate that AA deficiency may intensify oxidative damage leading to secondary effects on cellular structures and functions (5,33,36).
Recommendations for dietary intake of AA remain controversial. The increased demand for collagen synthesis in growing or injured individuals, or in diabetics with low plasma levels may require higher intakes of dietary AA. Studies with animals are difficult to extrapolate to humans as species requirement for AA most likely varies (4). As well, alterations in connective tissue by nutrition or other factors such as pharmaceuticals depend on whether they are of short-term or long-term duration. Age, type of nutritional alteration and specific tissue are also factors.
The Recommended Daily Allowance (RDA) for vitamin C was determined by the amount necessary to prevent scurvy and the amount where excess is eliminated from the body (by urine). The daily amount believed to prevent scurvy in an adult on a diet deficient in vitamin C is 10 mg/day. Considering depletion and turnover rates of whole body AA, the RDA was arbitrarily set at 60 mg/day for an adult male and female. However, by conventional reasoning, if the body requires higher levels of AA due to higher turnover rates, such as in disease or injury, then higher doses may be warranted and tolerated. Some proponents of increased vitamin C dosages use bowel tolerance to determine the maximal therapeutic dose.
Bowel tolerance is a method to determine body tolerance to AA. Theoretically, the sicker the body is, the more AA the body utilizes and can therefore tolerate higher doses. When plasma, intracellular and extracellular compartments are maximized, whole body tolerance is reached. Excess intake results in increased bowel activity. The levels where such symptoms appear vary individually with physiological status. During illness or injury, an individual may tolerate very high doses and experience no diarrhea. After the sickness or injury, the body does not require high doses and tolerance decreases, causing diarrhea. Many proponents claim that doses just below the bowel tolerance are most therapeutic. However, studies with such therapeutic doses have not been performed with human subjects. Nevertheless, research with animal models has shown that such therapeutic doses of AA resulted in reduced symptoms of osteoarthritis. Also, AA supplementation in surgical and non-surgical patients resulted in improved wound healing, reduced inflammation and improved recovery.
Vitamin B complex
The B vitamin complex is a large group of compounds with different structure and biological activity. They are usually found within the same food sources. The primary role of the B vitamins is cellular energy metabolism. Any deficit in cellular energy will have adverse effects on cellular function. Therefore, the B vitamins are essential in connective tissue metabolism.
Many of the B complex serve as cofactors in process of collagen and elastin cross-linking. Deficiencies in several of the B vitamins influence expression of collagen genes and induce decreased mechanical strength of repaired and remodeled tissue (32, 34,35).
Since most all B vitamins are found together in similar food groups, deficiencies of one singular vitamin is uncommon. However, deficiencies may exist if overall dietary intake is reduced. A mixture of all B vitamins should adequately provide for daily needs. Since most athletes supplement with a multivitamin product, intakes are generally at or above the RDA.
Vitamin A
Retinoids are a group of compounds of which some have vitamin A activity and others do not. Vitamin A is often referred to as retinol in much of the literature and will be used interchangeably here. Although carotenoids are commonly mistaken for vitamin A, only a fraction of them have any vitamin A activity. b -Carotene is the most significant because in the body it can be broken down into two retinol molecules and therefore supply vitamin A when needed. Retinol is stored in the liver and distributed to peripheral tissues by strict regulatory mechanisms and metabolized in several pathways (7).
Retinol is converted to retinoic acid inside cells and both are potent regulators of specific genes, including expression of fibronectin and type I procollagen (32,35). Other metabolites of retinol regulate cell differentiation and are associated with glycosaminoglycan (GAG), glycoprotein and proteoglycan (PG) synthesis. Although still unclear, the role of vitamin A in PG synthesis may be involved in sulfation of GAGs. Tissue from animals deficient in vitamin A typically displays decreased synthesis of highly sulfated GAGs (35).
Few in vivo studies exist documenting specific roles of retinoids in connective tissue, except for those studying wound healing in animal models. That rapidly growing tissues are sensitive to vitamin A deficiency is well known. Deficiency of other nutrients, such as zinc and protein, that assist in transport and metabolism of retinol may induce deficiency symptoms (8). Therefore, since retinol distribution from the liver is tightly regulated, functional deficiencies may result with normal vitamin A intake and stores. Additionally, extra-physiological doses of vitamin A may counteract the inhibitory effects of systemic corticosteroids on plasma retinol transport (32, 34).
Because vitamin A is fat-soluble, toxicity is also a concern in connective tissue metabolism. High levels may inhibit collagen synthesis, as seen in the skin, and increase catabolism of cartilage. This may be concentration dependent since excessively high levels affect ascorbate induced lipid peroxidation, which in turn inhibits AA-induced collagen synthesis (35,36).
Vitamin E
Vitamin E is a group of compounds comprising of two major classes: tocopherols and tocotrienols. The basic chemical structure in each class is similar with variations of substituents and confirmation resulting in different relative activity. For a complete discussion of these vitamers, readers are referred to a text on nutrition or medicinal chemistry. I use the term vitamin E in this article as a reference primarily to the tocopherols, as they have the greatest activity in the body.
Literature information on the role of vitamin E in connective tissue metabolism is controversial. The major function of vitamin E is as an antioxidant and in the maintenance of cell membrane integrity. Its role as an antioxidant is thought to require vitamin C and selenium. Although no specific disease of connective tissue can be attributed to vitamin E deficiency, it is no doubt needed for life and cell processes.
Animal model studies have shown that severe deficiency in vitamin E influence collagen cross-linking and an increase in susceptibility of insoluble collagen to degradation by proteinases (35). Conversely, excessive doses of vitamin E elicit effects similar to those of corticosteroids: inhibition of collagen synthesis and wound repair. Rats given supra-physiological doses of vitamin E exhibited less tensile strength in skin of healed wounds. Indeed, vitamin E may potentiate adverse effects of corticosteroids (34,35).
Vitamin E has exhibited anti-inflammatory effects in some animal models. As an antioxidant, vitamin E may protect lysosome membranes leading to a decrease in histamine and serotonin from mast cells during inflammation. However, studies show that the vitamin E has a preventative role rather than therapeutic. If sufficient vitamin E is present before inflammation response is initiated, the inflammatory phase may be shortened. Apparently, therapeutic administration (i.e. administration after the induction of inflammation) did not affect duration or progress of the inflammation phase (32). Thus perhaps optimal results may be seen only in individuals with degenerative joint conditions or with chronic inflammation.
Minerals
Minerals are required for normal cell function and several serve as cofactors in the many enzymatic processes involved in synthesis connective tissue macromolecules. Copper and manganese are critical cofactors for collagen and GAG synthesis and metabolism. Some recent research alludes to an increased role of manganese in synthesis of GAGs (10). However, a deficiency in these minerals is extremely rare. Some pharmaceuticals are known to negatively interact with some minerals. Nonetheless, defects in collagen synthesis are generally observed only at the lowest levels of dietary intake of most minerals. An athlete eating a diet with adequate protein and calories is likely to have normal levels of minerals.
Clinical evidence is largely lacking for effects of mineral deficiencies on connective tissue except for zinc. This mineral primarily acts as cofactor in many enzyme systems that regulate cell proliferation and growth and in immune integrity. Diminution of collagen synthesis and strength as well as impaired healing is seen in animal tissues with zinc deficiencies.
Controversy exists in whether supplemental zinc can accelerate healing above the normal rate. Several supplement companies in the sports marketing arena claim that most athletes are deficient in this mineral. However, total body assessment of zinc is not easily obtained and many published studies have erroneously relied on data interpretation from zinc plasma concentrations in humans (8,9,11,32). As well, most studies do not measure plasma concentration versus time to assess fluctuations.
Zinc exists in intracellular and extracellular pools and its exchange in the body is tightly regulated (12). Many factors influence tissue pool concentrations, such as absorption, oral contraceptives, and steroid therapy (11). Nonetheless, a well-nourished athlete with a healthy intake of animal protein, fruit, vegetables and a vitamin/mineral supplement is unlikely to be deficient in zinc. Populations who may exhibit deficiencies are the elderly, those with malabsorption, and lactovegetarians who consume large amounts of foods with phytates.
Pharmaceuticals
Nonsteroidal Anti-Inflammatory Drugs (NSAIDs)
NSAIDs, such as aspirin and ibuprofen, are routinely administered to treat connective tissue injuries. The anti-inflammatory and analgesic properties of these drugs are effectively employed for short-term relief. The immediate action of NSAIDs is due to decreased prostaglandin E2 (PGE2) synthesis by inhibition of cyclooxygenase (the rate-limiting enzyme involved in PGE2 synthesis). Invariably, the most common side effect is gastrointestinal (GI) upset, sometimes leading to peptic ulcers. Recent research has identified two isoforms of cyclooxygenase. The GI irritability of NSAIDs is due to their inhibition of Cox-l in the intestinal tract, whereas the therapeutic inflammatory effects are a result of inhibition of Cox-2 (13) New compounds are being developed to preferentially target Cox-2 and offer some relief of GI irritability (14,15).
Despite the efficacy of NSAIDs to reduce inflammation and pain, several in vitro and in vivo animal studies suggest that chronic use of some NSAIDs may promote degradation of articular cartilage. In vitro studies with several NSAIDs, such as aspirin, fenoprofen, and ibuprofen, reported inhibited net proteoglycan synthesis by chondrocytes in normal and osteoarthritic cartilage (16-18). Inhibition was concentration-dependant; however, effects were more profound in cartilage from osteoarthritic subjects. This suggests that cartilage degeneration in arthritic patients could be accelerated with NSAID treatment.
Consequently, avoidance or short-term use of NSAIDs is increasingly favored by care-providers in connective tissue injuries. Less-hazardous use of acetaminophen may be effective, although long-term use can be hepatotoxic. Most NSAID research has focused on articular cartilage with few studies examining effects on dense connective tissue (i.e. tendons and ligaments). NSAIDs may have an effect on early stages of dense tissue repair, although significant inhibition of healing has not been documented (20,21).
Corticosteroids
Anti-inflammatory steroids, such as cortisone, hydrocortisone and prednisone, may be administered systemically or by injection into connective tissue such as in the synovial cavity of a joint. They act by suppressing the immune response: preventing the migration of inflammatory cells and stabilizing the lysosomal membranes in cells thereby inhibiting the production of prostaglandins (24). In addition, corticosteroids also inhibit fibroblast proliferation and collagen and GAG synthesis resulting in compromised wound healing (23). Short-term intra- and periarticular administration of low-dose steroids has not been documented to cause serious complications in normal individuals (19). However, reports of tendon ruptures, bone necrosis, accelerated joint destruction, impaired wound healing and metabolic disturbances have been reported with prolonged usage (20).
Recalling the discussion of inflammation in Part I of this article series: its important role in connective tissue macromolecule metabolism. Lymphocytes and neutrophils (inflammatory cells) may directly influence macromolecule turnover and promote collagen deposition and PG synthesis (22). Arguably, inflammation is an integral part of the healing process. Thus, by their very actions as anti-inflammatories, corticosteroids and NSAIDs may delay or impair the repair and remodeling process. Instead, treatment of inflammation during injuries should be considered as “managed inflammation”: relying on alternative means for pain relief (acetaminophen, ice packs and reduced activity) during the acute inflammation phase, and proper subsequent treatment depending on the nature of the injury. Rheumatic arthritis and osteoarthritis, however, require specific treatment due to the chronic inflammation typically seen in these diseases.
Anabolic Steroids
Athletes have used anabolic/androgenic steroids for decades with the intent to enhance performance or increase muscle mass. Steroids (testosterone analogs) that athletes use vary in their anabolic and androgenic activity; they are referred to as anabolic steroids (AS) for sake of simplicity in this article. Although their primary activity is anabolic and androgenic, AS influence other cellular and tissue functions. Their impact on connective tissue is discussed based on the research that is available.
As expected, the studies of AS impact on connective tissue is conflicting due to many factors: methodology, lack of dependable information submitted by human subjects, poor control of variables (diet, training, other drug use, etc), and subjective bias. The most reliable information is studies on animal models; however, extrapolation to humans is limited for obvious reasons. Haupt comprehensively reviews the literature on athletic steroid use and connective tissue as well as some of the animal studies (25). He rightly comments; “Extrapolating from current research provides some insight, but whether anabolic steroid use is beneficial or not remains unclear.”
Much of the literature focusing on athletic AS use case studies reporting increased injury rate, especially with concomitant use of corticosteroids. Few mention the dosage levels used by many of these athletes, which are generally supra-physiological and for long durations. Although AS have a reputation within the athletic arena as promoting recovery from injuries, there are no human studies to support accelerated healing of connective tissue. Some of the animal studies suggest that short-term low-dose AS administration may increase the collagen fibril diameter and thus strength of new collagen (2930,30). Inhofe et al. demonstrated that a 6-week course of AS (at doses comparable to the typical athlete’s administration) produced a stiffer tendon in exercised rats that failed with less elongation and energy than in control groups (26). The ultrastructural changes in tendon morphology of the AS+exercise subjects varied with an insignificant trend towards larger fibril diameters. These results contrast those reported by other authors (27,28) who observed changes in collagen fibril crimp angle and fibril length.
Inhofe et al. also examined the biomechanical and ultrastructural changes at 6 weeks after cessation of AS administration. Since the observed differences in the AS+exercise group were eliminated at 12 weeks, apparently effects induced by AS are reversible with drug withdrawal. Based on these results, AS use may have accelerated the same changes in mechanical properties that ultimately occurred in the control groups. However, this has limited extrapolation to repairing connective tissues in humans.
Thus far, we have seen how caloric intake, macro- and micronutrients, and several pharmaceuticals can influence metabolism of connective tissue. The import of this information is ultimately on maintaining the integrity of our joints, ligaments and tendons through life and injury. As we have learned, normal repair and remodeling of injured tissue requires a symphony of numerous processes and nutritive constituents. The last part of this series will examine the most recent non-conventional and non-drug protection and treatment for connective tissue: glycosaminoglycans.

References
5. Okuda Y, Magahama M, Mizutani M, et al. Ascorbic acid prevents the inhibition of DNA synthesis induced by high glucose concentration in cultured human endothelial cells. Diabetes Res, 1991; 18:65-68.
6. Russell JE, Manske PR. Ascorbic acid requirement for optimal flexor tendon repair in vitro. J Orthop Res, 1991; 9:714-719.
7. Foye WO, Lemke TL, Williams DA, eds. In: Principles of Medicinal Chemistry, fourth ed. 1995. Williams and Wilkins pub.
8. Groff JL, Gropper SS, Hunt SM. In: Advanced Nutrition and Human Metabolism, second ed. 1995. West Publishing Co.
9. Dimitrov NV, MacDonald D, Malovrh MM. Bioavailability of micronutrients in humans. J Appl Nutr 1997; 49:56-65.
10. Leach RM. Role of manganese in mucopolysaccharide metabolism. Fed Proc 1971; 30:991.
11. Milne DB. Assessment of zinc and copper nutritional status in man. Nutr MD 1987; 13:1-2.
12. King MT, King JC, Tamura T, et al. Assessment of zinc status. J Nutr 1990; 120:1474-1479.
13. Hla T, Neilson K. Human cyclooxygenase-2 cDNA. Proc Natl Acad Sci USA, 1992; 89:7384-7388.
14. Siverstein FE. Improving the gastrointestinal safety of NSAIDs: the development of Misoprostol – from hypothesis to clinical practice. Dig Dis Sci 1998; 43:447-458.
15. Smith RL. Evaluation of clinical agents on articular chondrocyte metabolism in vitro. J Rheumatol 1998; 25:1871-1873.
16. Hugenberg ST, Brandt KD, Cole CA. Effect of sodium salicylate, aspirin, and ibuprofen on enzymes required by the chondrocyte for synthesis of chondroitin sulfate. J Rheumatol 1993; 20:2128-2133.
17. Anastassiades T, Chopra R, Law C, Wong E. In vitro supperssion of transforming growth factor-b induced stimulation of glycosaminoglycan synthesis by acetylsalicylic acid and its reversal by misoprostol. J Rheumatol 1998; 25:1962-1967.
18. Brandt KD. Effects of nonsteroidal anti-inflammatory drugs on chondrocyte metabolism in vitro and in vivo. Am J Med 1987; 83:29-34.
19. Caldwell JR. Intra-articular corticosteroids. Guide to selection and indications for use. Drugs 1996; 52:507-514.
20. Buckwalter JA, Woo SL-Y. Tissue effects of medications in sports injuries. In: Orthopaedic Sports Medicine: Principles and Practice, volume 1. DeLee JC and Drez DD, eds. 1994. WB Saunders Company.
21. Frank CB, Hart DA. Cellular response to loading. In: Sports-Induced Inflammation: Clinical and Basic Science Concepts, Leadbetter WB, Buckwalter JA, Gordon SL, eds. 1989. American Acadademy of Orthopaedic Surgeons.
22. Buckley A, Hill KE, Davidson JM. Collagen metabolism. In: Immunochemical Techniques: Chemotaxis and Inflammation. The study of repair processes in inflammation. Di Sabato G, ed. 1988. Academic Press, Inc.
23. Plotkin MB, Foss ML, Goldin B, Ellis DG. Dose-dependant effects of anti-inflammatory steroid injections on mechanical properties of rat tail tendons. Med Sci Sports 1976, 8:230-234.
24. Somani SM. Pharmacology in Exercise and Sports. 1996. CRC Press.
25. Haupt HA. The role of anabolic steroids as modifiers of sports-induced inflammation. In: Sports-Induced Inflammation: Clinical and Basic Science Concepts, Leadbetter WB, Buckwalter JA, Gordon SL, eds. 1989. American Acadademy of Orthopaedic Surgeons.
26. Inhofe PD, Grana WA, Egle D, et al. The effects of anabolic steroids on rat tendon. An ultrastructural, biomechanical, and biochemical analysis. Am J Sports Med 1995; 23:227-232.
27. Wood TO, Cooke PH, Goodship AE. The effect of exercise and anabolic steroids on the mechanical properties and crimp morphology of rat tendon. Am J Sports Med 1988; 16:153-158.
28. Michna H. Tendon injuries induced by exercise and anabolic steroids in experimental mice. Int Orthop 1987; 11:157-162.
29. Karpakka JA, Pesola MK, Takala TE. The effects of anabolic steroids on collagen synthesis in rat skeletal muscle and tendon. A preliminary report. Am J Sports Med 1992; 20:262-266.
30. Wright JK, Smith AJ, Cawston TE, Hazelman BL. The effect of the anabolic steroid, stanozol, on the production of procollagenase by human synovial and skin fibroblasts in vitro. Agents Actions 1989; 28:279-282.
31. Michna H. Collagen fibril dynamics in the anulus fibrosus induced by anabolic steroid hormone. Acta Anat (Basel) 1989; 135:12-16.
32. Bucci LR. Nutrition Applied to Injury Rehabilitation and Sports Medicine.1995. Human Kinetics Press.
33. Fisher E, McLennan SV, Tada H, et al. Interaction of ascorbic acid and glucose on production of collagen and proteoglycan by fibroblasts. Diabetes 1991; 40:371-376.
34. Ruberg RL. Role of nutrition in wound healing. Surg Cl No Am 1984; 4:705-714.
35. Tinker D, Rucker R. Role of selected nutrients in synthesis, accumulation, and chemical modification of connective tissue proteins. Phys Rev 1985; 65:607-657.
36. Berg RA, Kerr JS. Nutritional aspects of collagen metabolism. Annu Rev Nutr 1992; 369-390.
About the author
Elzi has spent the last several decades trying to determine where 'home' is: from New York, Maine, California, Oregon and now Texas. As well, her career has encompassed tool & die apprentice, forest ranger, assistant extension agent, mother, sheep and horse rancher, and mad research scientist. She has also been a competitive bodybuilder, but has found true joy in powerlifting. When Elzi is not playing fetch with her 1200 lb four-footed buddy, she is most happy in the gym and in a research lab.
Leave a Reply
You must be logged in to post a comment.