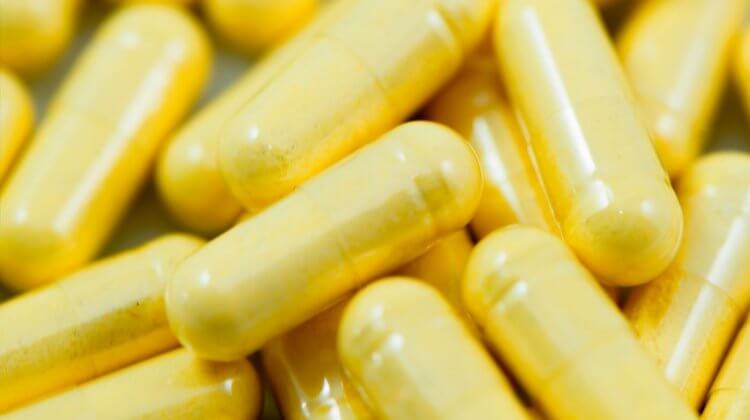
2,4-dinitrophenol (DNP) was a weight-loss drug that hit the shelves in the USA in the 1930s. It was used as a dye and in French ammunition factories as an ingredient for bombs during the First World War. Workers and soldiers exposed to the compound experienced various side effects, including of course, weight loss. Later, in the 1930s, the compound had attracted attention by researchers from Stanford. They had found it to be highly suitable for the purpose of increasing energy expenditure [1]. Indeed, their results in a small sample of subjects were quite remarkable. They reported an increase in energy expenditure of at least 40 % in most of the men. They display the metabolic rate and weight changes in one of the subjects in a figure:
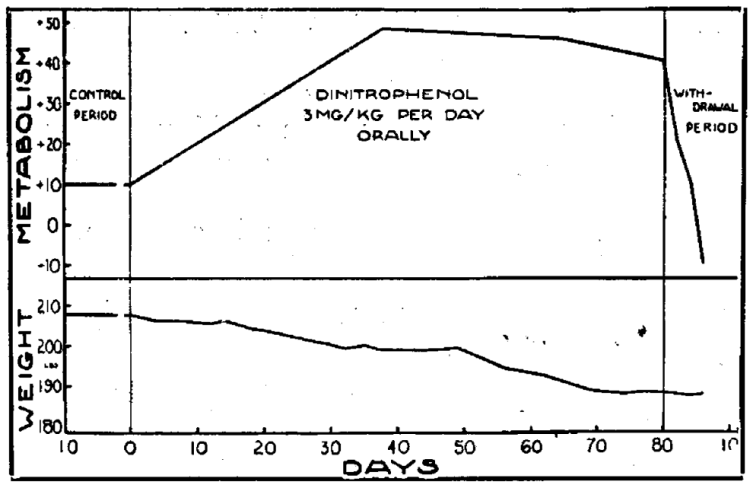
A later report by the same authors notes that they found an average increase in basal metabolic rate of 11 % for every 100 mg of DNP [2]. It got banned not much later, in 1938, as a result of some people dying from it.
But how on earth does this work? How does it increase the metabolic rate? DNP does this by acting as a so-called mitochondrial uncoupler. To understand what this means, I’ll provide a brief overview of how cells obtain the majority of their energy through something called oxidative phosphorylation. This process is what’s being ‘sabotaged’ by DNP. The increase in metabolic rate observed by increased thyroid hormone levels is also, to some part, the result of sabotaging this process—albeit achieved slightly differently.
Oxidative phosphorylation: obtaining energy from passing along electrons
It’s always a pleasure for me to cover some biochemistry in an article. I feel these underlying principles take away some of the magic from the effects of drugs, like DNP, and thereby provide a clearer picture of how things work. With some luck I might even, perhaps, interest some of you reading this into some further reading on the subject. Biochemistry and cell biology are extremely interesting fields of study.
The cells of your body continuously perform all sorts of functions in order to, essentially, keep you alive. A lot of these processes consume energy. This energy is, ultimately, derived from the foods you eat. Carbohydrates, fats and proteins, even alcohol, all have energy stored in their chemical bonds. It’s your body’s task to extract this energy and turn it into something useful. Much like how your car’s engine won’t run on crude oil, these cellular processes won’t run on macronutrients directly. Instead, most of these processes require their energy from a molecule called adenosine triphosphate (ATP)—just like how a car’s engine would require gasoline specifically.
Let’s have a look at how this would work for a glucose molecule, a carbohydrate. When a glucose molecule is used by a cell to yield ATP, it first undergoes a process called glycolysis. Glycolysis is a process of various enzymatic steps which will split the glucose molecule into 2 pyruvate molecules and produce 2 ATP molecules (among some other molecules). In a nutshell:
glucose -> 2 pyruvate + 2 ATP
That’s not much, yet. However, a process called oxidative phosphorylation will extract a lot more energy, i.e. ATP molecules, from the 2 resulting pyruvate molecules. And it’s precisely this process that’s being sabotaged by DNP.
Oxidative phosphorylation is a process that occurs in the mitochondria. So this is where the pyruvate is headed. The mitochondria are organelles of the cell that mainly deal with energy production. They’re microscopically small energy factories. They consist of an outer membrane and an inner membrane. The space between the outer and inner membrane is called the intermembrane space. The space encapsulated by the inner membrane is called the mitochondrial matrix. The inner membrane is folded into a characteristic way. These folds are called cristae. It looks like this:
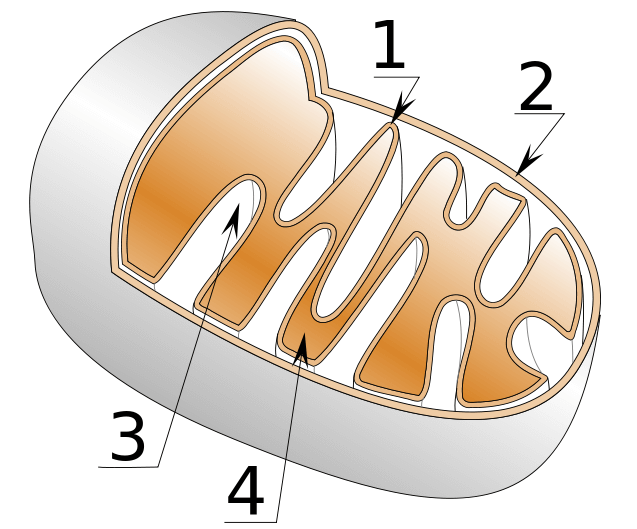
When pyruvate is inside the mitochondrial matrix, it gets converted into acetyl-CoA and subsequently undergoes a series of reactions which are collectively called the citric acid cycle or Krebs cycle. During this process, all the energy gets extracted from what was, originally, a pyruvate molecule. It gets burnt, oxidized. However, the energy hasn’t turned into ATP just yet. First, it’s transferred to the energy carriers NAD and FAD (and GTP, but I’m not gonna cover that). The energy carriers NAD and FAD will be the ones participating in the process called oxidative phosphorylation which follows the citric acid cycle.
The energy is stored in electron pairs that get donated to NAD and FAD. This reduces these molecules, as it’s called, yielding NADH and FADH2, respectively. What happens next is that NADH and FADH2 will hand over the electron pair to large protein complexes embedded in the inner membrane. This is the first step of oxidative phosphorylation. When these electron pairs are handed over to such a protein complex, some of the energy stored in it is used to pump a proton (H+) out of the mitochondrial matrix into the intermembrane space. This is super crucial and it will become clear why soon enough.
Subsequently, the electron pairs get handed over a couple of times from one complex to the next, each time peeling off some of the energy contained in them and using this energy to pump out a proton. With each step, the electrons reach a lower energy state. (They’re not directly handed over from one complex to the next, there are some intermediate proteins/molecules that ferry them around between these proton-pumping protein complexes.) And each time some of the energy that’s peeled off is harnessed to pump out a proton. Visualized using water wheels it would look something like this:
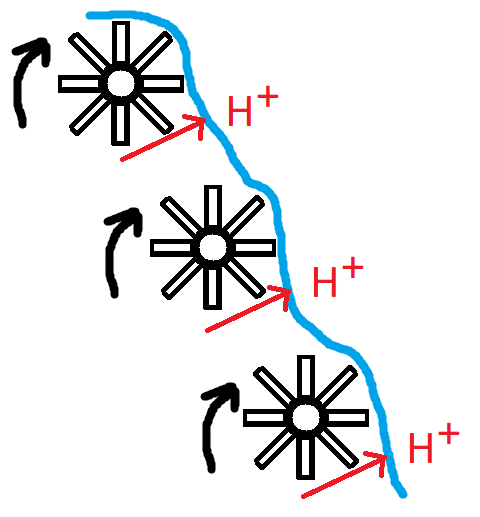
The final destination of the electrons is to combine with hydrogen and oxygen to form H2O, aka water. What this process of oxidative phosphorylation has established now, is an electrochemical gradient of protons. There will be a lower concentration of protons in the mitochondrial matrix compared to the intermembrane space. This gradient contains potential energy. Just as a water wheel will rotate with water moving downhill, a molecular machinery called ATP synthase starts to rotate with protons moving down their electrochemical gradient from the intermembrane space to the mitochondrial matrix. This energy is then harnessed to, finally, generate ATP by combining ADP with an inorganic phosphate group. And voila, that entire process of passing along the electrons, peeling off energy to pump out protons, and subsequently using the established proton gradient to synthesize ATP, is called oxidative phosphorylation.
To recap what we’ve covered, which has been quite a lot so far:
- Glucose gets split into two pyruvate molecules by glycolysis
- Pyruvate gets transported into the mitochondrial matrix to be converted to acetyl-CoA
- Acetyl-CoA gets oxidized, transferring its energy into the energy carriers NAD and FAD in their reduced forms NADH and FADH2 by accepting an electron pair
- These NADH and FADH2 molecules donate their electron pairs to a large protein complex embedded in the inner membrane, which then gets transferred on and on till it combines with hydrogen and oxygen to form water. With these transfers, some of the energy is harnessed to pump protons (H+) out of the mitochondrial matrix. This establishes an electrochemical gradient: low proton concentration inside the mitochondrial matrix, high proton concentration outside the mitochondrial matrix
- The flow of protons down their concentration gradient provides energy to ATP synthase to do its job and generate ATP
Now I hope you’re still with me, because we’re getting to the crux of the matter.
DNP lets protons leak back into the mitochondrial matrix
The section title says it all: DNP lets protons leak back into the mitochondrial matrix. All that elegant mitochondrial machinery to get those protons pumped out of the matrix so its backflow can be used to synthesize ATP? Yep, DNP sabotages that. DNP can function as a so-called protonophore. It can capture a proton in the intermembrane space, then move into the mitochondrial matrix, drop the proton off, and return to the intermembrane space. Just like that. This is not directed or anything: this works in both directions. However, as a result of the higher concentration of protons in the intermembrane space, and the lower proton concentration in the mitochondrial matrix, the net movement of protons by this process will be into the mitochondrial matrix. When DNP moves these protons, no ATP will be generated, as it doesn’t pass through the ATP synthase. Instead, it’s lost as heat.
Thyroid hormones can increase basal metabolic rate, partly, through a similar mechanism. Instead of moving the protons themselves, it’s thought that thyroid hormones increase the expression of uncoupling proteins [3, 4]. These are protein channels or transporters that are embedded in the inner membrane and allow protons to move from the intermembrane space to the mitochondrial matrix. Again, this will not be coupled to the synthesis of ATP, instead, the energy is lost as heat.
Additionally, DNP is thought to release calcium ions from both the mitochondria and the sarcoplasmic reticulum into the cytosol—which subsequently need to be pumped back. And, of course, pumping these ions back also costs energy. However, these effects are also thought to be a consequence of uncoupling of oxidative phosphorylation (by affecting the membrane potential) [5]. Finally, DNP is thought to interfere with uptake of inorganic phosphate groups into the mitochondrial matrix. These are required to synthesize ATP (as it’s formed by combining ADP with an inorganic phosphate group) [6].
References
- Cutting, W. C., H. Gr Mehrtens, and M. L. Tainter. “Actions and uses of dinitrophenol: promising metabolic applications.” Journal of the American Medical Association 101.3 (1933): 193-195.
- Tainter, M. L., A. B. Stockton, and W. C. Cutting. “Dinitrophenol in the treatment of obesity.” Journal of the American Medical Association 105.5 (1935): 332-337.
- Barbe, Pierre, et al. “Triiodothyronine‐mediated upregulation of UCP2 and UCP3 mRNA expression in human skeletal muscle without coordinated induction of mitochondrial respiratory chain genes.” The FASEB Journal 15.1 (2001): 13-15.
- de Lange, Pieter, et al. “Uncoupling protein-3 is a molecular determinant for the regulation of resting metabolic rate by thyroid hormone.” Endocrinology 142.8 (2001): 3414-3420.
- Hudman, Diane, et al. “The origin of calcium overload in rat cardiac myocytes following metabolic inhibition with 2, 4-dinitrophenol.” Journal of molecular and cellular cardiology 34.7 (2002): 859-871.
- Grundlingh, Johann, et al. “2, 4-dinitrophenol (DNP): a weight loss agent with significant acute toxicity and risk of death.” Journal of medical toxicology 7.3 (2011): 205-212.
About the author
Peter Bond is a scientific author with publications on anabolic steroids, the regulation of an important molecular pathway of muscle growth (mTORC1), and the dietary supplement phosphatidic acid. He is the author of several books in Dutch and English, including Book on Steroids and Bond's Dietary Supplements.
No replies yet
Loading new replies...
Join the full discussion at the MESO-Rx →