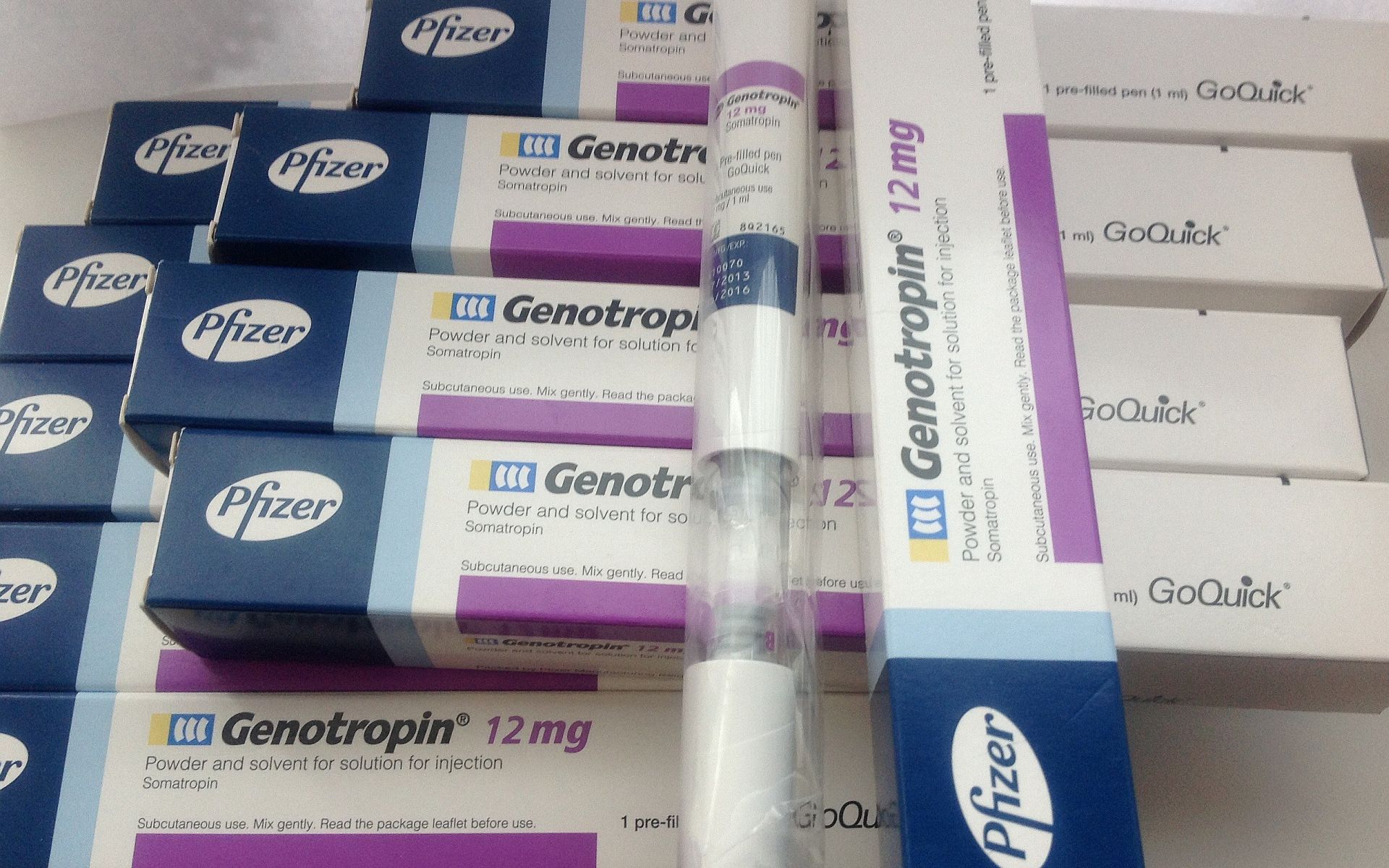
Pharmacokinetics, Pharmacodynamics and Negative Feedbacks
As I’ve touched on earlier, the liver is the major target for GH, with GH being the chief regulator of hepatic IGF-1 production. To accomplish this, GH binds in the liver with GHRs located within the extracellular domain of hepatocytes and subsequently stimulates the production of endocrine IGF-1 via gene transcription, utilizing the JAK-STAT signaling pathway. Further to this, GH administration has been shown to cause a rapid upregulation of IGF-1 mRNA within the liver [338].
Increased serum levels of IGF-1 also occur very quickly in the presence of a large bolus of rHGH. Significant elevations of IGF-1 are already observable by the 6-12 hour mark post injection [339]. These serum IGF-1 levels continue to increase until they reach their dose-dependent saturation point within 4-7 days, even when using extremely high doses that amount to 20-30 IUs per day of rHGH [340]. This particular saturation point turned out to be somewhere within the 700-800 ng/mL range, and seems to suggest endocrine levels of IGF-1 do have an upper ceiling in healthy adults. The exact mechanisms are yet to be elucidated, but are likely a result of the complex feedback loops which exist in the GH/IGF axis. Even those who feel strongly that elevating endocrine levels of IGF-1 is advantageous to maximizing hypertrophy potential should keep this in mind, as there is a point where more rHGH will simply not result in higher serum IGF-1 levels. I’ve attached the chart from the Tanaka study below:
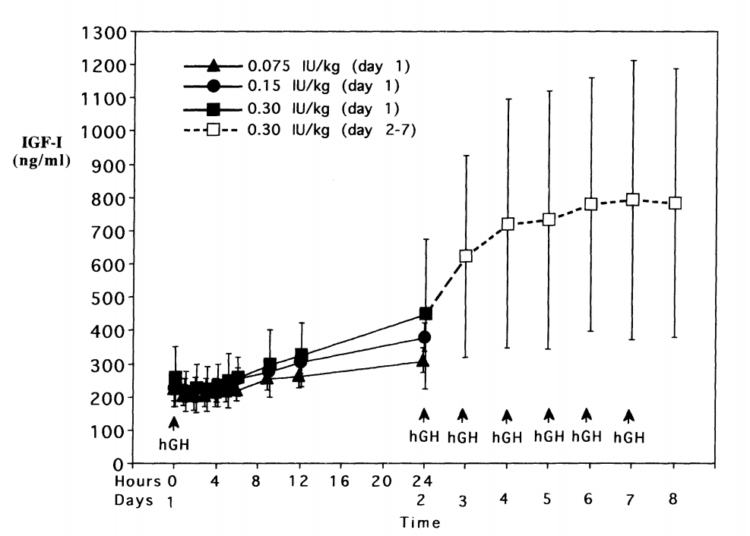
Now let’s spend a bit of time talking more about what autocrine IGF-1 does, and why it is a crucial mediator of the hypertrophy process, before getting back to further discussions on pharmacodynamics and pharmacokinetics. Signaling from the IGF-1 receptor is actually kind of unique in the sense that it uses two distinct pathways to stimulate either proliferation or differentiation [341-343]. This is quite interesting behavior, as no other growth factor family member has been shown to do this. Since proliferation and differentiation are opposing processes, it was originally difficult for researchers to understand how a single growth factor, via a single receptor, could send a signal which activated both [294]. Since those early discoveries were made, it has been further clarified that IGF-1 does not simultaneously perform these actions. Tests from various cell culture lines have demonstrated that the proliferative effects come first, lasting between 24-36 hours. It is only after this initial proliferative phase that myogenic differentiation occurs [344].
The IGF-1-mediated proliferative effects on myoblasts have been known since the 1970s, when it was first observed in rat liver cells [345]. This proliferative stimulation by IGF-1 results in an increase in cell number, protein levels, DNA synthesis, uptake of aminos, uptake of glucose, and suppression of proteolysis [346]. In human cell lines, IGF-1 has also been shown to increase the size of myotubes independent of whether myoblasts are actively proliferating, or if proliferation has ceased. It regulates myotube size by activating protein synthesis, inhibiting protein degradation, and inducing the fusion of reserve cells [347-348]. IGF’s ability to suppress proteolysis in skeletal muscle, the breakdown of proteins into aminos, has been demonstrated countless times over the years [349-352]. IGF-1 has also been shown to induce proliferation and differentiation of satellite cells into mature myocytes, as determined by an increase in the number of myofibers with centrally versus peripherally located nuclei [148,353-354].
The ability for autocrine IGF-1 to cause myoblast differentiation was actually a hybrid discovery that piggybacked off of studies from the 1960s demonstrating this effect occurred with high levels of insulin [355]. It was later shown that the IGFs are far more potent stimulators of myogenic differentiation than insulin and it was concluded that insulin really acts as an IGF-1 analog in this system [356-357]. The differentiation effects of autocrine IGF-1 are biphasic, with low concentrations progressively stimulating myoblast differentiation but very high concentrations showing all but ceased differentiating activity. The ceiling for differentiation to occur seems to be around 100 ng/mL for IGF-1 or 300 ng/mL for IGF-2 [358]. This is not caused by a switch to proliferation either, as there are no further increases in overall cell numbers observed [294]. It is possible that signaling molecules involved in the negative regulation of the myogenic system are increased, but this is speculative [359-360].
The administration of rHGH elevates local skeletal muscle IGF-1 mRNA expression in numerous cell, human, and animal models [127,150,361-364]. This happens quite quickly, within 60 minutes of a subcutaneous injection of rHGH, and peaks between the 6-12 hour mark [363]. In this particular cited animal model, doubling the dose of GH did not further increase IGF-1 mRNA levels, which suggests there is a ceiling effect with regard to how much GH is required to maximally stimulate local IGF-1 expression in skeletal muscle. We already have seen that IGF-1-mediated myocyte differentiation stops when local concentrations reach approximately 100 ng/mL but just how much GH is required to reach the IGF-1 mRNA expression saturation point?
45 replies
Loading new replies...
Join the full discussion at the MESO-Rx →