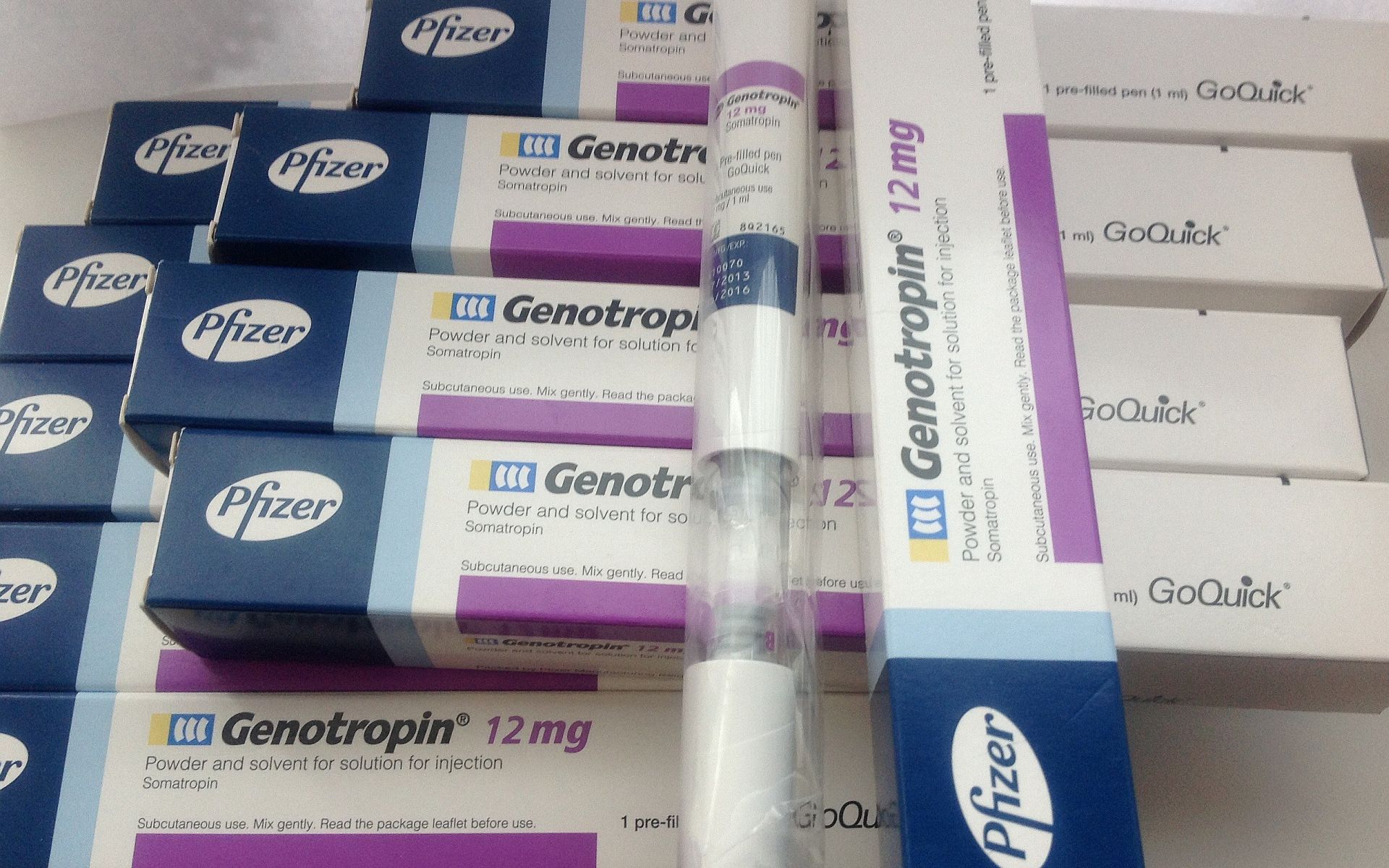
To provide dramatic examples of the importance that GH and IGF-1 have on postnatal growth, look no further than individuals with either mutations or disorders related to the GH/IGF axis. A detailed review is beyond the scope of this article but, generally speaking, disorders that suppress/inhibit the GH/IGF axis at a young age result in short stature while those that stimulate the GH/IGF axis result in gigantism [70-71].
The vast majority of GH’s growth promoting effects are mediated via IGF-1, however there are a handful of things that are GH-mediated, or IGF-independent. The most telling example of this would be present in animal models where double GH/IGF “knockout” mutants are more severely growth retarded than with either GH or IGF “knockout” alone [72]. But let’s instead look at some of the more specific effects that have been identified in human models.
The first is hepatic steatosis, also known as “fatty liver disease” [73]. It has been demonstrated in both humans with Laron Syndrome and animals with GHR suppression that this condition can still occur in the presence of suppressed IGF-1 [74]. Laron Syndrome provides some rather unique insights on the GH/IGF system due to the fact it is caused by a mutation in the GHR which results in significantly low levels of endocrine IGF-1, as GH has effectively been prevented from stimulating IGF-1 production.
Another GH-specific action is related to its ability to enhance ovarian preantral follicle development. In fact, GH has even been under investigation recently for its potential enhancements on female fertility. Results indicate that some groups using in vitro fertilization (IVF) do appear to benefit from the administration of GH [75-76]. GHR deficient animal models have also consistently shown lower numbers of primary preantral and antral follicles than their control littermates.
One of the more interesting, and earliest discovered, GH-specific actions is its ability to promote longitudinal bone growth via its effects on chondrocyte (cartilage cell) generation in the epiphyseal growth plate region [19-20,77-80]. GH actually has dual roles in its promotion of longitudinal bone growth; both the aforementioned effects on chondrocyte generation in the growth plate but also an IGF-1 mediated role in promoting chondrocyte hypertrophy [81]. It is worth noting that fully intact endocrine IGF-1 levels aren’t even a necessity when it comes to postnatal bone growth. In fact, as long as there are at least 10-20% levels of circulating endocrine IGF-1 present, the combination of autocrine IGF-1 and GH can still ensure normal postnatal bone growth is achieved [82]. This is likely due to the overlapping roles autocrine and endocrine IGF-1 have as it relates to this longitudinal bone growth [83].
And last, but not least, is likely going to be the most relevant GH-mediated effect to readers of this article. GH promotes increased rates of late-stage muscle cell fusion which may have the ability to increase muscle fiber size in a manner completely independent of IGF-1 upregulation [84]. Using a novel animal cell technique, researchers were able to demonstrate that GH promoted fusion of myoblasts with nascent myotubes without an increase in actual IGF-1 mRNA expression. Nascent myotubes are present in the later stages of muscle cell fusion [85] and GH was shown to increase the number of nuclei per myotube. This will be something we’ll go into further, and discuss why this can be particularly advantageous, as we dive deeper into GH and hypertrophy later in the article.
The Relationship Between GH Secretion and IGF-1
Growth hormone is well-known to increase levels of circulating IGF-1 as well as the synthesis of IGF-1 locally, in an autocrine manner. Both of these play critical roles in muscle mass regulation, so let’s take a moment to better understand how GH secretion leads to increased endocrine and autocrine levels of IGF-1.
The vast majority of growth hormone in healthy adults is secreted from the pituitary gland and, more specifically, by the somatotroph cells in the anterior lobe as mediated by the transcription factor Prophet of Pit-1 (PROP1) [86-87]. GH can also be synthesized locally in many tissues such as the brain, immune cells, mammary tissues, teeth, and placenta which are all outside the regulation of the pituitary [88]. This supports the idea that GH has autocrine roles in addition to its already well established endocrine roles.
GH is natively pulsatile in all species [89-90] and this secretory pattern plays a major physiological role in everything from its sexual dimorphic characteristics to IGF-1 mRNA expression, which we will discover more about as we move forward. Healthy young adult males secrete between 0.4mg – 0.5mg every 24 hours, and many of these secretions occur as “pulses within pulses” [91]. Normally, there are around 10-12 secretory bursts each day with men having a significantly more regular pulse pattern than women. In males, GH is secreted episodically, with the well-known large evening surge occurring near the onset of slow-wave sleep. Males also have less dramatic secretions which occur a few hours after consuming meals [92-94]. Females have higher inter-secretory trough levels, particularly in the follicular phase of menstruation, with more frequent GH pulses during the day and a significantly lower nocturnal pulse than males [95-96]. It is not entirely clear why this sexually dimorphic secretory pattern exists.
The secretion of GH is regulated in a very complex manner involving the participation of several neurotransmitters, as well as both hormonal and metabolic feedbacks. It is principally positively regulated by GHRH, aptly named growth hormone releasing hormone [97], and negatively regulated by SRIF, or Somatostatin. Both of these peptides are produced within the hypothalamus. In fact, in addition to its base role in hormone production, the hypothalamus is also consistently monitoring the GHRH/SRIF ratio and consequently controlling secretion by the pituitary [98].
In addition to its primary function of stimulating GH secretion, GHRH also plays an essential role in the proliferation and development of the aforementioned somatotroph cells. In fact, in environments with impaired or absent GHRH, anterior pituitary hypoplasia has been observed which is likely a result of somatotroph maldevelopment [99-100]. Humans who had GHRH suppressed by an antagonist demonstrated severely impaired pulsatile GH release as well as suppressed GH response to GHRH [101], so it is clearly a vital component within the GH/IGF axis.
SRIF, the main negative regulator of GH secretion, suppresses TSH. In addition, to a lesser degree, it also suppresses both prolactin and the adrenocorticotropic hormone [97]. All of these are well-known to have close relationships with the GH/IGF axis, so this is not entirely surprising. SRIF has a short half-life of around 2-3 minutes in serum and is then rapidly inactivated by tissue peptidases. During its active time, it suppresses not only spontaneous GH release but also GH response to all external stimuli including GHRH, hypoglycemia, arginine, and exercise just to name a few. Its suppressive effects seemingly are limited to both the magnitude of basal and pulsatile GH release, as it has not been shown to alter GH pulse frequency [102].
45 replies
Loading new replies...
Join the full discussion at the MESO-Rx →