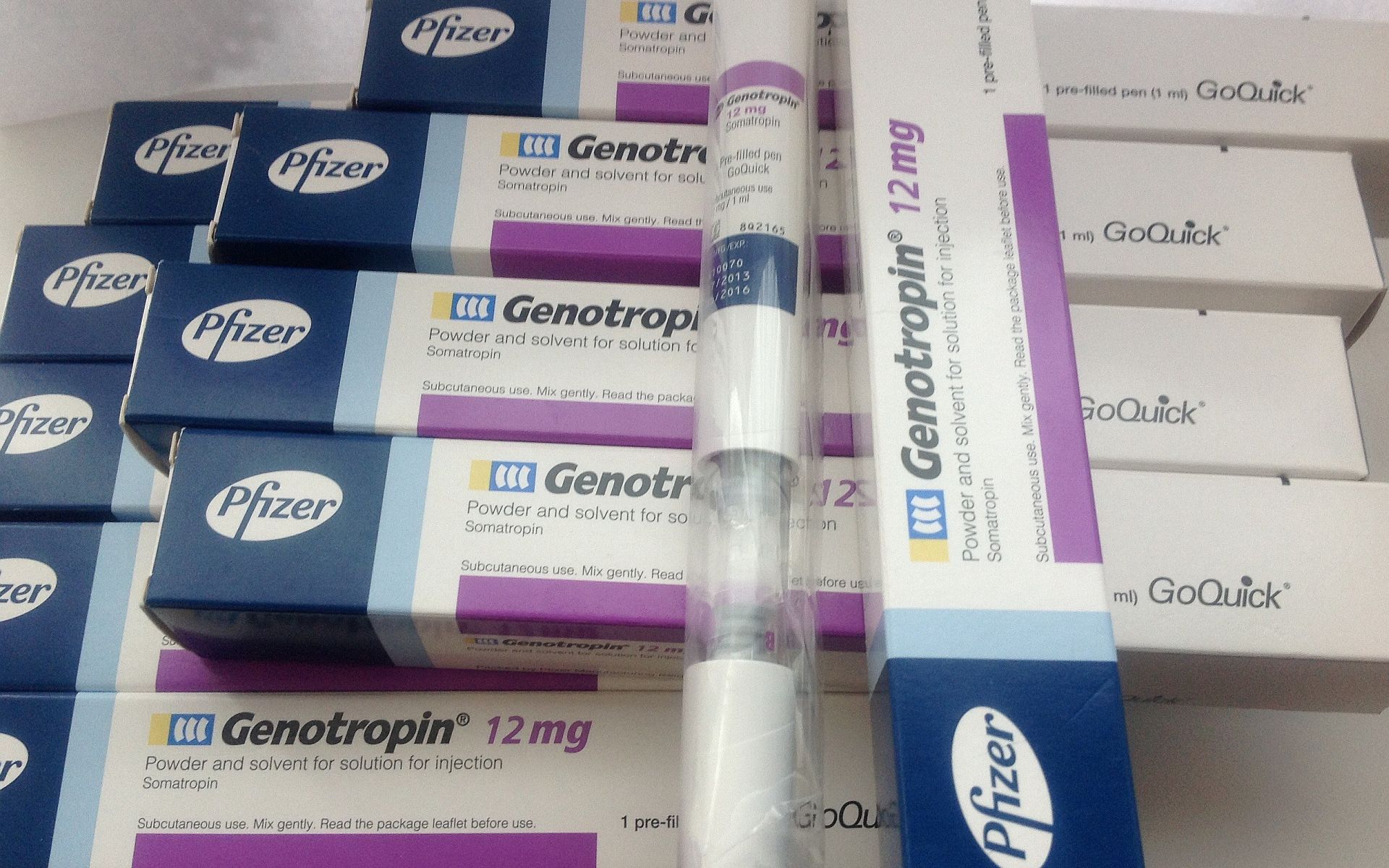
Decorin is a structural protein, residing primarily in the skeletal muscle extracellular matrix, and whose role is related to muscle growth and repair [222-223]. It was first shown that GH administration could directly increase decorin gene expression in animals a few decades ago [224]. It wasn’t until recently, however, that this effect was also shown to occur in recreationally trained human subjects [225]. In the more recent study, levels of decorin correlated strongly with those of PIIINP, which infers GH-mediated decorin stimulation may be involved in the bone collagen matrix assembly process. This effect is more pronounced in men than women and may be a byproduct of the higher IGF-1 levels seen in men, although this is speculative. The increased expression of decorin was not altered by the addition of testosterone, so this is an androgen-independent effect. After seeing the effects GH has on both collagen and decorin synthesis, it is becoming quite clear that the GH/IGF axis is far more important for strengthening the extracellular supportive matrix as opposed to directly contributing to skeletal muscle tissue growth.
Acute GH administration to healthy subjects has also been shown to cause increased mitochondrial ATP production and increased citrate synthase activity in skeletal muscle, with a higher abundance of muscle mRNAs encoding IGF-1 [226]. Not only could this be a contributing factor for why GH may have the ability to promote increased daily energy expenditure rates (an article for another day) but it may also be involved in the underlying shift to fat substrate fuel preference. Although, as we’ve reviewed earlier, the body of literature does not support this in practice, increased mitochondrial ATP production could play a role in aerobic capacity [227].
GH has been shown to promote the fusion of myoblasts with myotubes in cell models [84], an effect that is completely independent of local IGF-1 upregulation. To understand why this may be important, let’s deep-dive a bit further into the cellular factors involved in the hypertrophy of skeletal muscle. Skeletal muscle hypertrophy in humans relies on satellite cells, which are dormant cells located within myofibers, just under the basal lamina layer in the extracellular matrix [148]. Once these satellite cells are activated, often by exercise or muscle damage, they proliferate. After proliferation, these satellite cells migrate to sites of damage where they differentiate and fuse with existing myofibers which provides new nuclei for hypertrophy and repair [228]. It is still not entirely clear whether GH has a direct effect on the proliferation and differentiation of satellite cells [229-230]. It is also worth stating that what is seen in cell cultures may not be entirely indicative of what happens in vivo anyway due to various external factors which cannot be accounted for in lab conditions.
Continuing, there are two distinct stages of this myoblast fusion which take place [85]. The first would be the initial stage of differentiation where a subset of mononucleated cells fuse to form nascent myotubes (myoblast/myoblast fusion). This is followed by the second stage which involves additional available cells fusing with these nascent myotubes and where actual muscle growth occurs (myoblast/myotube fusion). It is within the latter stage where GH exerts its effects.
This is a pretty novel finding, but again, numerous trials on humans have failed to demonstrate a hypertrophic effect of GH in real-world conditions. So, we can probably infer from this that the effects GH has on the fusion of nascent myotubes does not translate directly into hypertrophy. However, what if we added another variable into the equation that could create an environment where enhanced satellite cell numbers existed, creating more raw materials for GH to work with [231]?
Introducing AAS
Unlike GH and IGF, the use of anabolic androgenic steroids (AAS) has a pronounced impact on both hypertrophy and strength. This has been well-known for decades and why they have been used and abused by athletes going as far back as their creation in the 1930s [232]. The AAS family consist of a potent group of synthetic compounds which are similar in chemical structure to testosterone and/or its 5alpha reduced derivative DHT. Various types of individual AAS compounds have been created over the years by first starting with the natural testosterone molecule and then manipulating it via the addition of an ethyl, methyl, hydroxyl, or benzyl group at one or more sites along its structure [233-234]. A few of the more easily recognized AAS variants include 17-alpha alkylated androgens, which are able to be orally administered, and 19-nortestosterone variants which remove the 19-methyl group from the testosterone molecule in an effort to increase its anabolic activity while simultaneously decreasing its aromatization potential. Also referred to as “19nor”, this family includes well-known AAS variants such as nandrolone and trenbolone.
Many of these compound discoveries came about as a result of a low-level desire to increase the anabolic characteristics of testosterone within muscle, while simultaneously lowering the androgenic side-effects natively inherent with the testosterone molecule [235]. Generally speaking, overall side-effect risks from chronic AAS use actually appears to be relatively low when compared to many socially acceptable drugs such as alcohol, tobacco, and various prescription medications [233,236]. Of course, use and abuse are mutually exclusive terms and abusing any substance tends to create a higher risk environment. Unless otherwise clearly noted, from this point forward, I will spend most of my time talking specifically about testosterone as it is the native male endogenous sex hormone as well as the most heavily studied androgen in the literature. For reference, healthy adult males produce between 14-77 mg/week of endogenous testosterone [435].
Testosterone is well-known to regulate muscle mass, and these testosterone-mediated increases in muscle mass are associated with fiber hypertrophy, as well as an increase in satellite cells and myonuclear number [231,237-240]. Skeletal muscle just so happens to be one of the most testosterone-responsive tissues, and circulating testosterone levels have been estimated to account for a large percentage (40-75%) of the gains in muscle mass observed in randomized control trials. If you recall from the last section, it has been demonstrated that GH possesses a very unique ability in that it can increase myoblast fusion during the hypertrophy process. Maximizing this capability relies upon having adequate numbers of available satellite cells. For now, just make a mental note as we’ll be circling back around to this in a bit.
Results consistently show that testosterone treatments result in dose-dependent increases in skeletal muscle mass and strength, independent of whether the subject groups include younger or older males [241-243]. Conversely, in trials with healthy young subjects where endogenous testosterone was artificially suppressed, strength and body composition both suffered significantly [244]. To reiterate our earlier point, testosterone-mediated increases in skeletal muscle mass are hypertrophy-based and not a result of either fiber transition (changing type one fibers to type two fibers or vice versa) or splitting [245]. Because of their unique and non-overlapping anabolic mechanisms, testosterone administration and resistance training have also shown synergistic, additive effects upon one another with regard to stimulating increases in muscle mass [246].
45 replies
Loading new replies...
Join the full discussion at the MESO-Rx →