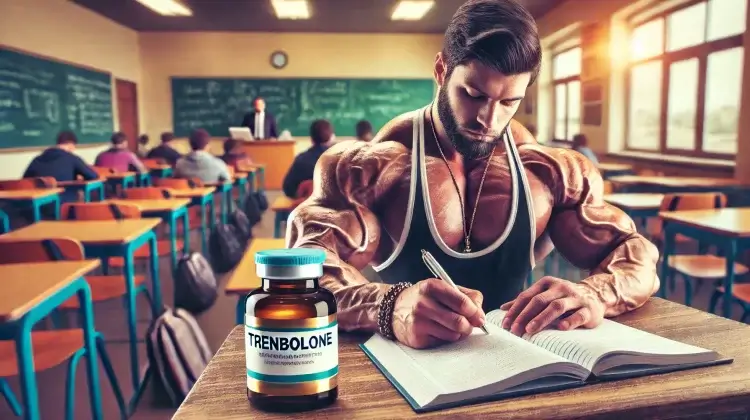
Estimated reading time: 9 minutes
Table of contents
Introduction
This is part one of an eleven-part series (1 / 11) that will delve into the unique features of anabolic-androgenic steroids (AAS) by compound or drug. It’s an exciting opportunity – (and to be frank, a fairly challenging one!) – to investigate the universe of commercially available AAS, and among the constellation of different classes and particular AAS, pick out their unique features or class effects for discussion.
Certainly, AAS features have more overlap than there are distinctions. Through the crudest lens, all AAS are basically related to testosterone – they all stimulate protein anabolism, male secondary sex characteristics (e.g., growth of body hair, voice deepening), and suppress luteinizing hormone (LH), follicle-stimulating hormone (FSH), and sex-hormone binding globulin (SHBG).
Through a more fine lens, however, distinctions bubble up to the surface of the ocean of commonalities. Some of these distinctions, or unique facets, might arise out of class effects. The FDA defines a drug class as a group of active moieties that share scientifically documented properties that are defined on the basis of any combination of three attributes of the active moiety:
- Mechanism of Action (MOA)
- Physiologic Effect (PE), and/or
- Chemical Structure (CS).
This series is ordered by classification by chemical structure (CS), grouping the AAS into the following chemical classes by structure, and further classifying by PE and/or CS where these distinctions are noteworthy:
- Trienes (Δ4,9,11 androgens)
- Heterocyclically-ringed (typically additional rings, e.g., furazane or pyrazole, attachments to the A-ring)
- Androst-4-ene-3-ones (classical androgens more like testosterone that tend to aromatize and either 5α- or 5β- reduce due to their C-4,5 double-bonded sterane structure unless another feature opposes these metabolic pathways)
- 5α-androstan-3-ones (nonaromatizable, non-5α-reducible androgens)
- Androst-1-ene-3-ones (“1-enes”; these C-1,2 double-bonded androgens generally present a decreased androgenic and to a lesser degree anabolic potency yielding a favorable anabolic-androgenic ratio)
- Androsta-1,4-dienes (“1,4-dienes”; more saturated A-ring androgens)
- 2-enes (highly unusual, lacking the 3-keto group, for which the O atom has a lone pair of electrons allowing its action as a H-bond acceptor, able to interact with polar or charged amino acids to form strong bond with the N-terminus/amino-group of the AR)
Further distinctions between AAS on the basis of drug effects are made. Drug effects are those effects of an AAS that arise not out of its class or common group structure but rather out of a particular characteristic – a MOA, PE, or CS – of the drug itself. For example, fluoxymesterone’s 11β-hydroxylation and its 9α-fluoronation promote its (antagonistic) binding to the glucocorticoid receptor (GR), a highly unusual feature that makes it act and look more like a glucocorticoid in some ways than an androgen, is not related to its androst-4-ene-3-one moieity – these features are responsible for fluoxymesterone’s drug effects, distinguished by a MOA (GR antagonism), PE (anticatabolism), and CS (a more glucocorticoid-like chemical structure).
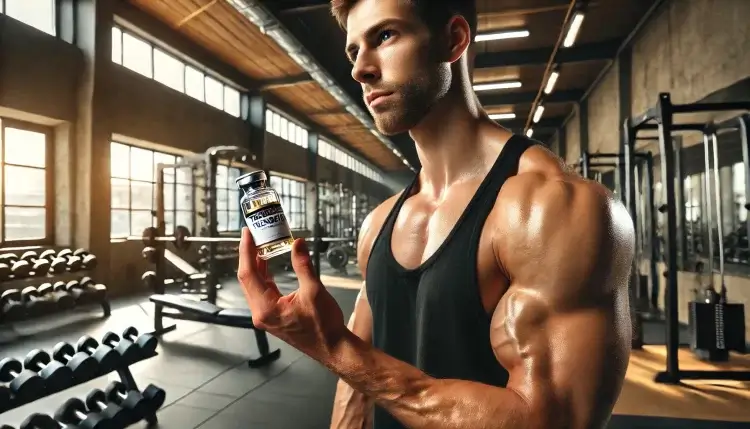
Trenbolone’s Unique Features
- Insulin sensitizing by reducing:
- GH pulse amplitude and duration (resulting in decreased serum IGF-I)
- PPARγ expression (anti-adipogenic, anti-hyperglycemic), and
- TAT activity (analogously to THG: “The Clear”) thereby decreasing gluconeogenesis and hepatic glucose output (lowering blood glucose).
- Anabolic effects by increasing the proliferative responsiveness of muscle satellite cells to IGF-I.
- Anticatabolic effects by decreasing muscle tissue GR number.
- Relative cardiac harm derives from inhibition of cortisol oxidation and exerting MR action, aggravating atherosclerosis via MR activation and inflammatory processes in the vascular endothelium.
Here, this author will begin with the triene, trenbolone, for a more nuanced assessment of its particularities that make it noteworthy – and indeed, make note:
Insulin sensitizing
Trenbolone decreases GH (endogenous pulse amplitude and duration)
Trenbolone reduces endogenous GH concentrations via a reduction in GH pulse amplitude and pulse duration. [1]. GH is secreted (provocatively during sleep, exercise stress, and starvation) pulsatilely – meaning that it is secreted as bursts (pulses). GH pulse amplitude refers to the height of “peaks” in GH concentrations and GH pulse duration refers to the time-course of each pulse (reduced pulse amplitude and duration therefore results in reduced blood GH area-under-the-curve).
Trenbolone’s well-known reduction to IGF-I is likely predominately due to its suppressing natural GH production.
While insulin resistance is generally understood to be “bad,” and insulin sensitivity “good” – for metabolic health, this is true – the manner in which GH provokes insulin resistance is by its increasing hormone-sensitive lipase activity (1 – 2 hr post-injection) [2], stimulating lipolysis, but also causing insulin resistance by increasing circulating free-fatty acids. [3].
Trenbolone decreases PPARγ expression (anti-adipogenic)
PPARγ is a peroxisome proliferator-activated receptor that controls the formation of new fat cells, fatty-acid uptake and storage, and therefore insulin sensitivity. [4]. Trenbolone decreases PPARγ mRNA expression during increased feeding. [5].
Adipogenesis refers to the process of generation (-genesis) of new fat cells (adipo-cytes). By decreasing PPARγ expression, trenbolone reduces body fat and circulating free-fatty acids that cause insulin resistance.
While the AR per se regulates insulin sensitivity, including by PPARγ reduction, trenbolone particularly reduces PPARγ expression, probably because of its potent activation of AR – “slicing” into it in a knife-like manner given its flatness and electrochemical properties – and this comports with Houtman that quantifies trenbolone’s AR transactivation potency as ≈ 4.5-fold that of testosterone’s. [12].
Trenbolone likely decreases TAT (tyrosine aminotransiferase) expression
Likely a class effect of the trienes (Δ4,9,11 androgens) – and so while not strictly unique to trenbolone, this feature is relegated to a small subset of commercially unavailable AAS like methyltrienolone (metribolone; R1881) – and importantly, THG (“The Clear”), to which notoriety is associated with Patrick Arnold’s BALCO Laboratories, a decrease in TAT expression in liver decreases gluconeogenesis & hepatic glucose output, thereby increasing insulin sensitivity. The TAT gene contains functional glucocorticoid response elements (GREs) in its promoter region (Muller et al, 1986; Becker et al, 1980) and its expression is directly regulated by glucocorticoids (Schmid et al, 1987). [6]. THG, and so by corollary the trienes including trenbolone, decrease it, increasing insulin sensitivity.
Anabolic
Trenbolone increases the proliferative responsiveness of skeletal muscle satellite cells to IGF-I
Skeletal muscle cells are uniquely multi-nucleated, and aggregate into fibers embedded in a matrix of collagen. Muscle injury triggers muscle repair (myogenesis), that partly overlaps with muscle remodeling (hypertrophy). In both processes, resistance training causes some damage to these muscle fibers by mechanical loading. When these muscle fibers are damaged, populations of pluripotent satellite cells that residing along muscle fibers (at the basal lamina) differentiate into single nucleated myoblasts that are capable of dividing and fusing with each other and existing myofibers to form new skeletal muscle tissue. [8]. Cells, including muscle, are regulated by the cell cycle: proliferation is the stage at which the number of cells are actually increased, and so increasing the proliferative responsiveness of satellite cells to IGF-I results in more new muscle being built in the presence of a given concentration of IGF-I.
Trenbolone potently increases the proliferative responsiveness of skeletal muscle satellite cells to IGF-I. [9].
![The effect of [IGF-I 10 ng/mL]...on total cell number in cultures from trenbolone-treated satellite cells (myotube nuclear density reflecting satellite cell proliferation). [9].](https://thinksteroids.com/wp-content/uploads/2024/06/trenbolone-increase-proliferative-response-to-IGF1-750x472.png)
The effect of [IGF-I 10 ng/mL]…on total cell number in cultures from trenbolone-treated satellite cells (myotube nuclear density reflecting satellite cell proliferation). [9].
![Effect of serum from control and trenbolone-treated rats on cell proliferation in muscle satellite cell cultures. [9].](https://thinksteroids.com/wp-content/uploads/2024/06/trenbolone-satellite-cells-750x498.png)
Effect of serum from control and trenbolone-treated rats on cell proliferation in muscle satellite cell cultures. [9].
Anticatabolic
Trenbolone decreases GR (glucocorticoid receptor) expression in muscle
Glucocorticoids like cortisol are catabolic steroids that decrease protein synthesis by affecting rates of translation. Trenbolone suppresses expression of glucocorticoid receptor (GR) mRNA in skeletal muscle to achieve a tissue-selective anticatabolism. [7]. Net muscle protein balance is the difference between muscle protein synthesis and muscle protein breakdown (net muscle = MPS – MPB). As such, trenbolone acts not only to increase the MPS side of the equation by increasing muscle expression of IGF-I and IGF-IEb (IGF-IEb mRNA expression, 3.6-fold increase & IGF-I a 5-fold increase) and by increasing myosin heavy chain (MHC) expression, but it uniquely suppresses GR number (upon which glucocorticoids like cortisol act to catabolize muscle tissue by binding as ligands), as well as suppressing expression of MuRF1 and atrogin-1 mRNA (more than testosterone), other pathways of muscle catabolism, to reduce MPB. [7].
Atherosclerotic and pro-inflammatory
Trenbolone likely antagonizes MR (mineralocorticoid receptor)
Impaired corticosteroid metabolism and activation of minerolocorticoid receptor (MR) has been associated with cardiovascular disease (Briet and chiffrin, 2010; Hadoke et al., 2009; Lastra et al., 2010). [10].
Antagonism of the MR inhibits the aldosterone-induced absorption of sodium ion (Na⁺) in the kidneys, collecting duct principal cells (Rafestin-Oblin et al., 2002), and affecting Na⁺ transport in the bladder. [11]. The principal consequences of MR antagonism are electrolyte disturbances (consequences of impaired aldosterone action) and the development of hypertension and cardiovascular disease.
Trenbolone, likely by acting analogously to the research steroid metribolone, antagonizes the MR, and so exerts atherosclerosis (plaque buildup in arteries) and inflammation, harmful to the heart.
References
[1] Hayden, J. M., Bergen, W. G., & Merkel, R. A. (1992). Skeletal muscle protein metabolism and serum growth hormone, insulin, and cortisol concentrations in growing steers implanted with estradiol-17β, trenbolone acetate, or estradiol-17β plus trenbolone acetate2. Journal of Animal Science, 70(7), 2109–2119. doi:10.2527/1992.7072109x
[2] Wee, J., Charlton, C., Simpson, H., Jackson, N. C., Shojaee-Moradie, F., Stolinski, M., … Umpleby, A. M. (2005). GH secretion in acute exercise may result in post-exercise lipolysis. Growth Hormone & IGF Research, 15(6), 397–404. doi:10.1016/j.ghir.2005.08.003
[3] Møller, N., & Jørgensen, J. O. L. (2009). Effects of Growth Hormone on Glucose, Lipid, and Protein Metabolism in Human Subjects. Endocrine Reviews, 30(2), 152–177. doi:10.1210/er.2008-0027
[4] Montaigne, D., Butruille, L., Staels, B. (2021). PPAR control of metabolism and cardiovascular functions. Nature Reviews Cardiology, 1–15.
[5] Chung, K. Y., Baxa, T. J., Parr, S. L., Luqué, L. D., & Johnson, B. J. (2012). Administration of estradiol, trenbolone acetate, and trenbolone acetate/estradiol implants alters adipogenic and myogenic gene expression in bovine skeletal muscle. Journal of Animal Science, 90(5), 1421–1427. doi:10.2527/jas.2010-3496
[6] Friedel, A., Geyer, H., Kamber, M., Laudenbach-Leschowsky, U., Schänzer, W., Thevis, M., … Diel, P. (2006). Tetrahydrogestrinone is a potent but unselective binding steroid and affects glucocorticoid signalling in the liver. Toxicology Letters, 164(1), 16–23. doi:10.1016/j.toxlet.2005.11.006
[7] Ye, F., McCoy, S. C., Ross, H. H., Bernardo, J. A., Beharry, A. W., Senf, S. M., … Borst, S. E. (2014). Transcriptional regulation of myotrophic actions by testosterone and trenbolone on androgen-responsive muscle. Steroids, 87, 59–66. doi:10.1016/j.steroids.2014.05.024
[8] Herbst, K. L., & Bhasin, S. (2004). Testosterone action on skeletal muscle. Current Opinion in Clinical Nutrition and Metabolic Care, 7(3), 271–277. doi:10.1097/00075197-200405000-00006
[9] Thompson SH, Boxhorn LK, Kong WY, Allen RE. Trenbolone alters the responsiveness of skeletal muscle satellite cells to fibroblast growth factor and insulin-like growth factor I. Endocrinology. 1989 May;12 4(5):2110-7. doi: 10.1210/endo-124-5-2110
[10] Furstenberger, C., Vuorinen, A., Da Cunha, T., Kratschmar, D. V., Saugy, M., Schuster, D., & Odermatt, A. (2012). The Anabolic Androgenic Steroid Fluoxymesterone Inhibits 11 -Hydroxysteroid Dehydrogenase 2-Dependent Glucocorticoid Inactivation. Toxicological Sciences, 126(2), 353–361. doi:10.1093/toxsci/kfs022
[11] Takeda AN, Pinon GM, Bens M, Fagart J, Rafestin-Oblin ME, Vandewalle A. The synthetic androgen methyltrienolone (R1881) acts as a potent antagonist of the mineralocorticoid receptor. Mol Pharmacol. 2007 Feb;71(2):473-82. doi: 10.1124/mol.106.031112
[12] Houtman, C. J., Sterk, S. S., van de Heijning, M. P. M., Brouwer, A., Stephany, R. W., van der Burg, B., & Sonneveld, E. (2009). Detection of anabolic androgenic steroid abuse in doping control using mammalian reporter gene bioassays. Analytica Chimica Acta, 637(1-2), 247–258. doi:10.1016/j.aca.2008.09.037
About the author
Type-IIx is a physique coach, author, and researcher. Bolus: A Practical Guide and Reference for recombinant Human Growth Hormone Use will be his first published textbook, anticipated for release in early 2023. Ampouletude.com will be Type-IIx's base of operations for coaching services and publications. Type-IIx is proud to be a contributing writer to MesoRx, his home forum, where he is a regular poster.
51 replies
Loading new replies...
Join the full discussion at the MESO-Rx →